«First planet» redirects here. For other systems of numbering planets, see Planet § History.
![]() Mercury in true color (by MESSENGER in 2008) | ||||||||||||
Designations | ||||||||||||
---|---|---|---|---|---|---|---|---|---|---|---|---|
Pronunciation | (![]() | |||||||||||
Adjectives | Mercurian ,[1] Mercurial [2] | |||||||||||
Orbital characteristics[5] | ||||||||||||
Epoch J2000 | ||||||||||||
Aphelion |
| |||||||||||
Perihelion |
| |||||||||||
Semi-major axis |
| |||||||||||
Eccentricity | 0.205630[3] | |||||||||||
Orbital period (sidereal) |
| |||||||||||
Orbital period (synodic) | 115.88 d[3] | |||||||||||
Average orbital speed | 47.36 km/s[3] | |||||||||||
Mean anomaly | 174.796° | |||||||||||
Inclination |
| |||||||||||
Longitude of ascending node | 48.331° | |||||||||||
Argument of perihelion | 29.124° | |||||||||||
Satellites | None | |||||||||||
Physical characteristics | ||||||||||||
Mean diameter | 4880 km | |||||||||||
Mean radius |
| |||||||||||
Flattening | 0.0009[3] | |||||||||||
Surface area |
| |||||||||||
Volume |
| |||||||||||
Mass |
| |||||||||||
Mean density | 5.427 g/cm3[6] | |||||||||||
Surface gravity |
| |||||||||||
Moment of inertia factor | 0.346±0.014[9] | |||||||||||
Escape velocity | 4.25 km/s[6] | |||||||||||
Synodic rotation period | 176 d[10] | |||||||||||
Sidereal rotation period |
| |||||||||||
Equatorial rotation velocity | 10.892 km/h (3.026 m/s) | |||||||||||
Axial tilt | 2.04′ ± 0.08′ (to orbit)[9] (0.034°)[3] | |||||||||||
North pole right ascension |
| |||||||||||
North pole declination | 61.45°[3] | |||||||||||
Albedo |
| |||||||||||
Temperature | 437 K (164 °C) (blackbody temperature)[13] | |||||||||||
| ||||||||||||
Apparent magnitude | −2.48 to +7.25[15] | |||||||||||
Angular diameter | 4.5–13″[3] | |||||||||||
Atmosphere[3][16][17] | ||||||||||||
Surface pressure | trace (≲ 0.5 nPa) | |||||||||||
Composition by volume |
| |||||||||||
Mercury is the smallest planet in the Solar System and the closest to the Sun. Its orbit around the Sun takes 87.97 Earth days, the shortest of all the Sun’s planets. It is named after the Roman god Mercurius (Mercury), god of commerce, messenger of the gods, and mediator between gods and mortals, corresponding to the Greek god Hermes (Ἑρμῆς). Like Venus, Mercury orbits the Sun within Earth’s orbit as an inferior planet; its apparent distance from the Sun as viewed from Earth never exceeds 28°. This proximity to the Sun means the planet can only be seen near the western horizon after sunset or the eastern horizon before sunrise, usually in twilight. At this time, it may appear as a bright star-like object, but is more difficult to observe than Venus. From Earth, the planet telescopically displays the complete range of phases, similar to Venus and the Moon, which recurs over its synodic period of approximately 116 days. Due to its synodic proximity to Earth, Mercury is most often the closest planet to Earth, with Venus periodically taking this role.[18][19]
Mercury rotates in a way that is unique in the Solar System. It is tidally locked with the Sun in a 3:2 spin–orbit resonance,[20] meaning that relative to the fixed stars, it rotates on its axis exactly three times for every two revolutions it makes around the Sun.[a][21] As seen from the Sun, in a frame of reference that rotates with the orbital motion, it appears to rotate only once every two Mercurian years. An observer on Mercury would therefore see only one day every two Mercurian years.
Mercury’s axis has the smallest tilt of any of the Solar System’s planets (about 1⁄30 degree). Its orbital eccentricity is the largest of all known planets in the Solar System;[b] at perihelion, Mercury’s distance from the Sun is only about two-thirds (or 66%) of its distance at aphelion. Mercury’s surface appears heavily cratered and is similar in appearance to the Moon’s, indicating that it has been geologically inactive for billions of years. Having almost no atmosphere to retain heat, it has surface temperatures that vary diurnally more than on any other planet in the Solar System, ranging from 100 K (−173 °C; −280 °F) at night to 700 K (427 °C; 800 °F) during the day across the equatorial regions.[22] The polar regions are constantly below 180 K (−93 °C; −136 °F). The planet has no natural satellites.
Two spacecraft have visited Mercury: Mariner 10 flew by in 1974 and 1975; and MESSENGER, launched in 2004, orbited Mercury over 4,000 times in four years before exhausting its fuel and crashing into the planet’s surface on April 30, 2015.[23][24][25] The BepiColombo spacecraft is planned to arrive at Mercury in 2025.
Nomenclature
The ancients knew Mercury by different names depending on whether it was an evening star or a morning star. By about 350 BC, the ancient Greeks had realized the two stars were one.[26] They knew the planet as Στίλβων Stilbōn, meaning «twinkling», and Ἑρμής Hermēs, for its fleeting motion,[27] a name that is retained in modern Greek (Ερμής Ermis).[28] The Romans named the planet after the swift-footed Roman messenger god, Mercury (Latin Mercurius), which they equated with the Greek Hermes, because it moves across the sky faster than any other planet.[26][29] The astronomical symbol for Mercury is a stylized version of Hermes’ caduceus; a Christian cross was added in the 16th century: .[30][31]
Physical characteristics
Mercury is one of four terrestrial planets in the Solar System, and is a rocky body like Earth. It is the smallest planet in the Solar System, with an equatorial radius of 2,439.7 kilometres (1,516.0 mi).[3] Mercury is also smaller—albeit more massive—than the largest natural satellites in the Solar System, Ganymede and Titan. Mercury consists of approximately 70% metallic and 30% silicate material.[32]
Internal structure
Mercury’s internal structure and magnetic field
Mercury appears to have a solid silicate crust and mantle overlying a solid, iron sulfide outer core layer, a deeper liquid core layer, and a solid inner core.[33][34] The planet’s density is the second highest in the Solar System at 5.427 g/cm3, only slightly less than Earth’s density of 5.515 g/cm3.[3] If the effect of gravitational compression were to be factored out from both planets, the materials of which Mercury is made would be denser than those of Earth, with an uncompressed density of 5.3 g/cm3 versus Earth’s 4.4 g/cm3.[35] Mercury’s density can be used to infer details of its inner structure. Although Earth’s high density results appreciably from gravitational compression, particularly at the core, Mercury is much smaller and its inner regions are not as compressed. Therefore, for it to have such a high density, its core must be large and rich in iron.[36]
The radius of Mercury’s core is estimated to be 2,020 ± 30 km (1,255 ± 19 mi), based on interior models constrained to be consistent with the value of the moment of inertia factor given in the infobox.[9][37] Hence, Mercury’s core occupies about 57% of its volume; for Earth this proportion is 17%. Research published in 2007 suggests that Mercury has a molten core.[38][39] Surrounding the core is a 500–700 km (310–430 mi) mantle consisting of silicates.[40][41] Based on data from the Mariner 10 and MESSENGER missions, in addition to Earth-based observation, Mercury’s crust is estimated to be 35 km (22 mi) thick.[42][43] However, this model may be an overestimate and the crust could be 26 ± 11 km (16.2 ± 6.8 mi) thick based on an Airy isostacy model.[44] One distinctive feature of Mercury’s surface is the presence of numerous narrow ridges, extending up to several hundred kilometers in length. It is thought that these were formed as Mercury’s core and mantle cooled and contracted at a time when the crust had already solidified.[45][46][47]
Mercury’s core has a higher iron content than that of any other major planet in the Solar System, and several theories have been proposed to explain this. The most widely accepted theory is that Mercury originally had a metal–silicate ratio similar to common chondrite meteorites, thought to be typical of the Solar System’s rocky matter, and a mass approximately 2.25 times its current mass.[48] Early in the Solar System’s history, Mercury may have been struck by a planetesimal of approximately 1/6 Mercury’s mass and several thousand kilometers across.[48] The impact would have stripped away much of the original crust and mantle, leaving the core behind as a relatively major component.[48] A similar process, known as the giant impact hypothesis, has been proposed to explain the formation of the Moon.[48]
Alternatively, Mercury may have formed from the solar nebula before the Sun’s energy output had stabilized. It would initially have had twice its present mass, but as the protosun contracted, temperatures near Mercury could have been between 2,500 and 3,500 K and possibly even as high as 10,000 K.[49] Much of Mercury’s surface rock could have been vaporized at such temperatures, forming an atmosphere of «rock vapor» that could have been carried away by the solar wind.[49] A third hypothesis proposes that the solar nebula caused drag on the particles from which Mercury was accreting, which meant that lighter particles were lost from the accreting material and not gathered by Mercury.[50]
Each hypothesis predicts a different surface composition, and two space missions have been tasked with making observations of this composition. The first MESSENGER, which ended in 2015, found higher-than-expected potassium and sulfur levels on the surface, suggesting that the giant impact hypothesis and vaporization of the crust and mantle did not occur because said potassium and sulfur would have been driven off by the extreme heat of these events.[51] BepiColombo, which will arrive at Mercury in 2025, will make observations to test these hypotheses.[52] The findings so far would seem to favor the third hypothesis; however, further analysis of the data is needed.[53]
Surface geology
Mercury’s surface is similar in appearance to that of the Moon, showing extensive mare-like plains and heavy cratering, indicating that it has been geologically inactive for billions of years. It is more heterogeneous than the surface of Mars or the Moon, both of which contain significant stretches of similar geology, such as maria and plateaus.[54] Albedo features are areas of markedly different reflectivity, which include impact craters, the resulting ejecta, and ray systems. Larger albedo features correspond to higher reflectivity plains.[55] Mercury has dorsa (also called «wrinkle-ridges»), Moon-like highlands, montes (mountains), planitiae (plains), rupes (escarpments), and valles (valleys).[56][57]
MASCS spectrum scan of Mercury’s surface by MESSENGER
The planet’s mantle is chemically heterogeneous, suggesting the planet went through a magma ocean phase early in its history. Crystallization of minerals and convective overturn resulted in layered, chemically heterogeneous crust with large-scale variations in chemical composition observed on the surface. The crust is low in iron but high in sulfur, resulting from the stronger early chemically reducing conditions than is found in the other terrestrial planets. The surface is dominated by iron-poor pyroxene and olivine, as represented by enstatite and forsterite, respectively, along with sodium-rich plagioclase and minerals of mixed magnesium, calcium, and iron-sulfide. The less reflective regions of the crust are high in carbon, most likely in the form of graphite.[58]
Names for features on Mercury come from a variety of sources. Names coming from people are limited to the deceased. Craters are named for artists, musicians, painters, and authors who have made outstanding or fundamental contributions to their field. Ridges, or dorsa, are named for scientists who have contributed to the study of Mercury. Depressions or fossae are named for works of architecture. Montes are named for the word «hot» in a variety of languages. Plains or planitiae are named for Mercury in various languages. Escarpments or rupēs are named for ships of scientific expeditions. Valleys or valles are named for abandoned cities, towns, or settlements of antiquity.[59]
Impact basins and craters
Mercury was heavily bombarded by comets and asteroids during and shortly following its formation 4.6 billion years ago, as well as during a possibly separate subsequent episode called the Late Heavy Bombardment that ended 3.8 billion years ago.[60] Mercury received impacts over its entire surface during this period of intense crater formation,[57] facilitated by the lack of any atmosphere to slow impactors down.[61] During this time Mercury was volcanically active; basins were filled by magma, producing smooth plains similar to the maria found on the Moon.[62][63] One of the most unusual craters is Apollodorus, or «the Spider», which hosts a serious of radiating troughs extending outwards from its impact site.[64]
Craters on Mercury range in diameter from small bowl-shaped cavities to multi-ringed impact basins hundreds of kilometers across. They appear in all states of degradation, from relatively fresh rayed craters to highly degraded crater remnants. Mercurian craters differ subtly from lunar craters in that the area blanketed by their ejecta is much smaller, a consequence of Mercury’s stronger surface gravity.[65] According to International Astronomical Union rules, each new crater must be named after an artist who was famous for more than fifty years, and dead for more than three years, before the date the crater is named.[66]
Overhead view of Caloris Basin
Perspective view of Caloris Basin – high (red); low (blue)
The largest known crater is Caloris Planitia, or Caloris Basin, with a diameter of 1,550 km.[67] The impact that created the Caloris Basin was so powerful that it caused lava eruptions and left a concentric mountainous ring ~2 km tall surrounding the impact crater. The floor of the Caloris Basin is filled by a geologically distinct flat plain, broken up by ridges and fractures in a roughly polygonal pattern. It is not clear whether they are volcanic lava flows induced by the impact or a large sheet of impact melt.[65]
At the antipode of the Caloris Basin is a large region of unusual, hilly terrain known as the «Weird Terrain». One hypothesis for its origin is that shock waves generated during the Caloris impact traveled around Mercury, converging at the basin’s antipode (180 degrees away). The resulting high stresses fractured the surface.[68] Alternatively, it has been suggested that this terrain formed as a result of the convergence of ejecta at this basin’s antipode.[69]
Tolstoj basin is along the bottom of this image of Mercury’s limb
Overall, 46 impact basins have been identified.[70] A notable basin is the 400 km wide, multi-ring Tolstoj Basin that has an ejecta blanket extending up to 500 km from its rim and a floor that has been filled by smooth plains materials. Beethoven Basin has a similar-sized ejecta blanket and a 625 km diameter rim.[65] Like the Moon, the surface of Mercury has likely incurred the effects of space weathering processes, including solar wind and micrometeorite impacts.[71]
Plains
There are two geologically distinct plains regions on Mercury.[65][72] Gently rolling, hilly plains in the regions between craters are Mercury’s oldest visible surfaces,[65] predating the heavily cratered terrain. These inter-crater plains appear to have obliterated many earlier craters, and show a general paucity of smaller craters below about 30 km in diameter.[72]
Smooth plains are widespread flat areas that fill depressions of various sizes and bear a strong resemblance to the lunar maria. Unlike lunar maria, the smooth plains of Mercury have the same albedo as the older inter-crater plains. Despite a lack of unequivocally volcanic characteristics, the localisation and rounded, lobate shape of these plains strongly support volcanic origins.[65] All the smooth plains of Mercury formed significantly later than the Caloris basin, as evidenced by appreciably smaller crater densities than on the Caloris ejecta blanket.[65]
Compressional features
One unusual feature of Mercury’s surface is the numerous compression folds, or rupes, that crisscross the plains. These also exist on the moon, but are much more prominent on Mercury.[73] As Mercury’s interior cooled, it contracted and its surface began to deform, creating wrinkle ridges and lobate scarps associated with thrust faults. The scarps can reach lengths of 1000 km and heights of 3 km.[74] These compressional features can be seen on top of other features, such as craters and smooth plains, indicating they are more recent.[75] Mapping of the features has suggested a total shrinkage of Mercury’s radius in the range of ~1 to 7 km.[76] Most activity along the major thrust systems probably ended about 3.6–3.7 billion years ago.[77] Small-scale thrust fault scarps have been found, tens of meters in height and with lengths in the range of a few km, that appear to be less than 50 million years old, indicating that compression of the interior and consequent surface geological activity continue to the present.[74][76]
Volcanism
Picasso crater — the large arc-shaped pit located on the eastern side of its floor are postulated to have formed when subsurface magma subsided or drained, causing the surface to collapse into the resulting void.
There is evidence for pyroclastic flows on Mercury from low-profile shield volcanoes.[78][79][80] 51 pyroclastic deposits have been identified,[81] where 90% of them are found within impact craters.[81] A study of the degradation state of the impact craters that host pyroclastic deposits suggests that pyroclastic activity occurred on Mercury over a prolonged interval.[81]
A «rimless depression» inside the southwest rim of the Caloris Basin consists of at least nine overlapping volcanic vents, each individually up to 8 km in diameter. It is thus a «compound volcano».[82] The vent floors are at least 1 km below their brinks and they bear a closer resemblance to volcanic craters sculpted by explosive eruptions or modified by collapse into void spaces created by magma withdrawal back down into a conduit.[82] Scientists could not quantify the age of the volcanic complex system but reported that it could be on the order of a billion years.[82]
Surface conditions and exosphere
Composite of the north pole of Mercury, where NASA confirmed the discovery of a large volume of water ice, in permanently dark craters that are found there.[83]
The surface temperature of Mercury ranges from 100 to 700 K (−173 to 427 °C; −280 to 800 °F)[22] at the most extreme places: 0°N, 0°W, or 180°W. It never rises above 180 K at the poles,[14] due to the absence of an atmosphere and a steep temperature gradient between the equator and the poles. The subsolar point reaches about 700 K during perihelion (0°W or 180°W), but only 550 K at aphelion (90° or 270°W).[84]
On the dark side of the planet, temperatures average 110 K.[14][85]
The intensity of sunlight on Mercury’s surface ranges between 4.59 and 10.61 times the solar constant (1,370 W·m−2).[86]
Although the daylight temperature at the surface of Mercury is generally extremely high, observations strongly suggest that ice (frozen water) exists on Mercury. The floors of deep craters at the poles are never exposed to direct sunlight, and temperatures there remain below 102 K, far lower than the global average.[87] This creates a cold trap where ice can accumulate. Water ice strongly reflects radar, and observations by the 70-meter Goldstone Solar System Radar and the VLA in the early 1990s revealed that there are patches of high radar reflection near the poles.[88] Although ice was not the only possible cause of these reflective regions, astronomers think it was the most likely.[89]
The icy regions are estimated to contain about 1014–1015 kg of ice,[90] and may be covered by a layer of regolith that inhibits sublimation.[91] By comparison, the Antarctic ice sheet on Earth has a mass of about 4×1018 kg, and Mars’s south polar cap contains about 1016 kg of water.[90] The origin of the ice on Mercury is not yet known, but the two most likely sources are from outgassing of water from the planet’s interior or deposition by impacts of comets.[90]
Mercury is too small and hot for its gravity to retain any significant atmosphere over long periods of time; it does have a tenuous surface-bounded exosphere[92] containing hydrogen, helium, oxygen, sodium, calcium, potassium and others[16][17] at a surface pressure of less than approximately 0.5 nPa (0.005 picobars).[3] This exosphere is not stable—atoms are continuously lost and replenished from a variety of sources. Hydrogen atoms and helium atoms probably come from the solar wind, diffusing into Mercury’s magnetosphere before later escaping back into space. Radioactive decay of elements within Mercury’s crust is another source of helium, as well as sodium and potassium. MESSENGER found high proportions of calcium, helium, hydroxide, magnesium, oxygen, potassium, silicon and sodium. Water vapor is present, released by a combination of processes such as: comets striking its surface, sputtering creating water out of hydrogen from the solar wind and oxygen from rock, and sublimation from reservoirs of water ice in the permanently shadowed polar craters. The detection of high amounts of water-related ions like O+, OH−, and H3O+ was a surprise.[93][94] Because of the quantities of these ions that were detected in Mercury’s space environment, scientists surmise that these molecules were blasted from the surface or exosphere by the solar wind.[95][96]
Sodium, potassium and calcium were discovered in the atmosphere during the 1980–1990s, and are thought to result primarily from the vaporization of surface rock struck by micrometeorite impacts[97] including presently from Comet Encke.[98] In 2008, magnesium was discovered by MESSENGER.[99] Studies indicate that, at times, sodium emissions are localized at points that correspond to the planet’s magnetic poles. This would indicate an interaction between the magnetosphere and the planet’s surface.[100]
On November 29, 2012, NASA confirmed that images from MESSENGER had detected that craters at the north pole contained water ice. MESSENGER‘s principal investigator Sean Solomon is quoted in The New York Times estimating the volume of the ice to be large enough to «encase Washington, D.C., in a frozen block two and a half miles deep».[83]
According to NASA, Mercury is not a suitable planet for Earth-like life. It has a surface boundary exosphere instead of a layered atmosphere, extreme temperatures, and high solar radiation. It is unlikely that any living beings can withstand those conditions.[101] Some parts of the subsurface of Mercury may have been habitable, and perhaps life forms, albeit likely primitive microorganisms, may have existed on the planet.[102][103][104]
Magnetic field and magnetosphere
Graph showing relative strength of Mercury’s magnetic field
Despite its small size and slow 59-day-long rotation, Mercury has a significant, and apparently global, magnetic field. According to measurements taken by Mariner 10, it is about 1.1% the strength of Earth’s. The magnetic-field strength at Mercury’s equator is about 300 nT.[105][106] Like that of Earth, Mercury’s magnetic field is dipolar.[100] Unlike Earth’s, Mercury’s poles are nearly aligned with the planet’s spin axis.[107] Measurements from both the Mariner 10 and MESSENGER space probes have indicated that the strength and shape of the magnetic field are stable.[107]
It is likely that this magnetic field is generated by a dynamo effect, in a manner similar to the magnetic field of Earth.[108][109] This dynamo effect would result from the circulation of the planet’s iron-rich liquid core. Particularly strong tidal heating effects caused by the planet’s high orbital eccentricity would serve to keep part of the core in the liquid state necessary for this dynamo effect.[40][110]
Mercury’s magnetic field is strong enough to deflect the solar wind around the planet, creating a magnetosphere. The planet’s magnetosphere, though small enough to fit within Earth,[100] is strong enough to trap solar wind plasma. This contributes to the space weathering of the planet’s surface.[107] Observations taken by the Mariner 10 spacecraft detected this low energy plasma in the magnetosphere of the planet’s nightside. Bursts of energetic particles in the planet’s magnetotail indicate a dynamic quality to the planet’s magnetosphere.[100]
During its second flyby of the planet on October 6, 2008, MESSENGER discovered that Mercury’s magnetic field can be extremely «leaky». The spacecraft encountered magnetic «tornadoes» – twisted bundles of magnetic fields connecting the planetary magnetic field to interplanetary space – that were up to 800 km wide or a third of the radius of the planet. These twisted magnetic flux tubes, technically known as flux transfer events, form open windows in the planet’s magnetic shield through which the solar wind may enter and directly impact Mercury’s surface via magnetic reconnection[111] This also occurs in Earth’s magnetic field. The MESSENGER observations showed the reconnection rate is ten times higher at Mercury, but its proximity to the Sun only accounts for about a third of the reconnection rate observed by MESSENGER.[111]
Orbit, rotation, and longitude
Orbit of Mercury (2006)
Animation of Mercury’s and Earth’s revolution around the Sun
Mercury has the most eccentric orbit of all the planets in the Solar System; its eccentricity is 0.21 with its distance from the Sun ranging from 46,000,000 to 70,000,000 km (29,000,000 to 43,000,000 mi). It takes 87.969 Earth days to complete an orbit. The diagram illustrates the effects of the eccentricity, showing Mercury’s orbit overlaid with a circular orbit having the same semi-major axis. Mercury’s higher velocity when it is near perihelion is clear from the greater distance it covers in each 5-day interval. In the diagram, the varying distance of Mercury to the Sun is represented by the size of the planet, which is inversely proportional to Mercury’s distance from the Sun. This varying distance to the Sun leads to Mercury’s surface being flexed by tidal bulges raised by the Sun that are about 17 times stronger than the Moon’s on Earth.[112] Combined with a 3:2 spin–orbit resonance of the planet’s rotation around its axis, it also results in complex variations of the surface temperature.[32]
The resonance makes a single solar day (the length between two meridian transits of the Sun) on Mercury last exactly two Mercury years, or about 176 Earth days.[113]
Mercury’s orbit is inclined by 7 degrees to the plane of Earth’s orbit (the ecliptic), the largest of all eight known solar planets.[114] As a result, transits of Mercury across the face of the Sun can only occur when the planet is crossing the plane of the ecliptic at the time it lies between Earth and the Sun, which is in May or November. This occurs about every seven years on average.[115]
Mercury’s axial tilt is almost zero,[116] with the best measured value as low as 0.027 degrees.[117] This is significantly smaller than that of Jupiter, which has the second smallest axial tilt of all planets at 3.1 degrees. This means that to an observer at Mercury’s poles, the center of the Sun never rises more than 2.1 arcminutes above the horizon.[117]
At certain points on Mercury’s surface, an observer would be able to see the Sun peek up a little more than two-thirds of the way over the horizon, then reverse and set before rising again, all within the same Mercurian day.[c] This is because approximately four Earth days before perihelion, Mercury’s angular orbital velocity equals its angular rotational velocity so that the Sun’s apparent motion ceases; closer to perihelion, Mercury’s angular orbital velocity then exceeds the angular rotational velocity. Thus, to a hypothetical observer on Mercury, the Sun appears to move in a retrograde direction. Four Earth days after perihelion, the Sun’s normal apparent motion resumes.[32] A similar effect would have occurred if Mercury had been in synchronous rotation: the alternating gain and loss of rotation over revolution would have caused a libration of 23.65° in longitude.[118]
For the same reason, there are two points on Mercury’s equator, 180 degrees apart in longitude, at either of which, around perihelion in alternate Mercurian years (once a Mercurian day), the Sun passes overhead, then reverses its apparent motion and passes overhead again, then reverses a second time and passes overhead a third time, taking a total of about 16 Earth-days for this entire process. In the other alternate Mercurian years, the same thing happens at the other of these two points. The amplitude of the retrograde motion is small, so the overall effect is that, for two or three weeks, the Sun is almost stationary overhead, and is at its most brilliant because Mercury is at perihelion, its closest to the Sun. This prolonged exposure to the Sun at its brightest makes these two points the hottest places on Mercury. Maximum temperature occurs when the Sun is at an angle of about 25 degrees past noon due to diurnal temperature lag, at 0.4 Mercury days and 0.8 Mercury years past sunrise.[119] Conversely, there are two other points on the equator, 90 degrees of longitude apart from the first ones, where the Sun passes overhead only when the planet is at aphelion in alternate years, when the apparent motion of the Sun in Mercury’s sky is relatively rapid. These points, which are the ones on the equator where the apparent retrograde motion of the Sun happens when it is crossing the horizon as described in the preceding paragraph, receive much less solar heat than the first ones described above.[120]
Mercury attains inferior conjunction (nearest approach to Earth) every 116 Earth days on average,[3] but this interval can range from 105 days to 129 days due to the planet’s eccentric orbit. Mercury can come as near as 82,200,000 km (0.549 astronomical units; 51.1 million miles) to Earth, and that is slowly declining: The next approach to within 82,100,000 km (51 million mi) is in 2679, and to within 82,000,000 km (51 million mi) in 4487, but it will not be closer to Earth than 80,000,000 km (50 million mi) until 28,622.[121] Its period of retrograde motion as seen from Earth can vary from 8 to 15 days on either side of inferior conjunction. This large range arises from the planet’s high orbital eccentricity.[32] Essentially because Mercury is closest to the Sun, when taking an average over time, Mercury is most often the closest planet to the Earth,[18][19] and—in that measure—it is the closest planet to each of the other planets in the Solar System.[122][123][124][d]
Longitude convention
The longitude convention for Mercury puts the zero of longitude at one of the two hottest points on the surface, as described above. However, when this area was first visited, by Mariner 10, this zero meridian was in darkness, so it was impossible to select a feature on the surface to define the exact position of the meridian. Therefore, a small crater further west was chosen, called Hun Kal, which provides the exact reference point for measuring longitude.[125][126] The center of Hun Kal defines the 20° west meridian. A 1970 International Astronomical Union resolution suggests that longitudes be measured positively in the westerly direction on Mercury.[127] The two hottest places on the equator are therefore at longitudes 0° W and 180° W, and the coolest points on the equator are at longitudes 90° W and 270° W. However, the MESSENGER project uses an east-positive convention.[128]
Spin-orbit resonance
After one orbit, Mercury has rotated 1.5 times, so after two complete orbits the same hemisphere is again illuminated.
For many years it was thought that Mercury was synchronously tidally locked with the Sun, rotating once for each orbit and always keeping the same face directed towards the Sun, in the same way that the same side of the Moon always faces Earth. Radar observations in 1965 proved that the planet has a 3:2 spin-orbit resonance, rotating three times for every two revolutions around the Sun. The eccentricity of Mercury’s orbit makes this resonance stable—at perihelion, when the solar tide is strongest, the Sun is nearly still in Mercury’s sky.[129]
The rare 3:2 resonant tidal locking is stabilized by the variance of the tidal force along Mercury’s eccentric orbit, acting on a permanent dipole component of Mercury’s mass distribution.[130] In a circular orbit there is no such variance, so the only resonance stabilized in such an orbit is at 1:1 (e.g., Earth–Moon), when the tidal force, stretching a body along the «center-body» line, exerts a torque that aligns the body’s axis of least inertia (the «longest» axis, and the axis of the aforementioned dipole) to point always at the center. However, with noticeable eccentricity, like that of Mercury’s orbit, the tidal force has a maximum at perihelion and therefore stabilizes resonances, like 3:2, ensuring that the planet points its axis of least inertia roughly at the Sun when passing through perihelion.[130]
The original reason astronomers thought it was synchronously locked was that, whenever Mercury was best placed for observation, it was always nearly at the same point in its 3:2 resonance, hence showing the same face. This is because, coincidentally, Mercury’s rotation period is almost exactly half of its synodic period with respect to Earth. Due to Mercury’s 3:2 spin-orbit resonance, a solar day lasts about 176 Earth days.[32] A sidereal day (the period of rotation) lasts about 58.7 Earth days.[32]
Simulations indicate that the orbital eccentricity of Mercury varies chaotically from nearly zero (circular) to more than 0.45 over millions of years due to perturbations from the other planets.[32][131]
This was thought to explain Mercury’s 3:2 spin-orbit resonance (rather than the more usual 1:1), because this state is more likely to arise during a period of high eccentricity.[132]
However, accurate modeling based on a realistic model of tidal response has demonstrated that Mercury was captured into the 3:2 spin-orbit state at a very early stage of its history, within 20 (more likely, 10) million years after its formation.[133]
Numerical simulations show that a future secular orbital resonant perihelion interaction with Jupiter may cause the eccentricity of Mercury’s orbit to increase to the point where there is a 1% chance that the orbit will be destabilized in the next five billion years. If this happens, Mercury may fall into the Sun, collide with Venus, be ejected from the Solar System, or even disrupt the rest of the inner Solar System.[134][135]
Advance of perihelion
In 1859, the French mathematician and astronomer Urbain Le Verrier reported that the slow precession of Mercury’s orbit around the Sun could not be completely explained by Newtonian mechanics and perturbations by the known planets. He suggested, among possible explanations, that another planet (or perhaps instead a series of smaller «corpuscules») might exist in an orbit even closer to the Sun than that of Mercury, to account for this perturbation.[136] (Other explanations considered included a slight oblateness of the Sun.) The success of the search for Neptune based on its perturbations of the orbit of Uranus led astronomers to place faith in this possible explanation, and the hypothetical planet was named Vulcan, but no such planet was ever found.[137]
The perihelion precession of Mercury is 5,600 arcseconds (1.5556°) per century relative to Earth, or 574.10±0.65 arcseconds per century[138] relative to the inertial ICRF. Newtonian mechanics, taking into account all the effects from the other planets, predicts a precession of 5,557 arcseconds (1.5436°) per century.[138] In the early 20th century, Albert Einstein’s general theory of relativity provided the explanation for the observed precession, by formalizing gravitation as being mediated by the curvature of spacetime. The effect is small: just 42.98 arcseconds per century for Mercury; it therefore requires a little over twelve million orbits for a full excess turn. Similar, but much smaller, effects exist for other Solar System bodies: 8.62 arcseconds per century for Venus, 3.84 for Earth, 1.35 for Mars, and 10.05 for 1566 Icarus.[139][140]
Observation
Image mosaic by Mariner 10, 1974
Mercury’s apparent magnitude is calculated to vary between −2.48 (brighter than Sirius) around superior conjunction and +7.25 (below the limit of naked-eye visibility) around inferior conjunction.[15] The mean apparent magnitude is 0.23 while the standard deviation of 1.78 is the largest of any planet. The mean apparent magnitude at superior conjunction is −1.89 while that at inferior conjunction is +5.93.[15] Observation of Mercury is complicated by its proximity to the Sun, as it is lost in the Sun’s glare for much of the time. Mercury can be observed for only a brief period during either morning or evening twilight.[141]
But in some cases Mercury can better be observed in daylight with a telescope when the position is known because it is higher in the sky and less atmospheric effects affect the view of the planet. When proper safety precautions are taken to prevent inadvertently pointing the telescope at the Sun (and thus blinding the user), Mercury can be viewed as close as 4° to the Sun when near superior conjunction when it is almost at its brightest.
Mercury can, like several other planets and the brightest stars, be seen during a total solar eclipse.[142]
Like the Moon and Venus, Mercury exhibits phases as seen from Earth. It is «new» at inferior conjunction and «full» at superior conjunction. The planet is rendered invisible from Earth on both of these occasions because of its being obscured by the Sun,[141] except its new phase during a transit.
Mercury is technically brightest as seen from Earth when it is at a full phase. Although Mercury is farthest from Earth when it is full, the greater illuminated area that is visible and the opposition brightness surge more than compensates for the distance.[143] The opposite is true for Venus, which appears brightest when it is a crescent, because it is much closer to Earth than when gibbous.[143][144]
False-color map showing the maximum temperatures of the north polar region
Nonetheless, the brightest (full phase) appearance of Mercury is an essentially impossible time for practical observation, because of the extreme proximity of the Sun. Mercury is best observed at the first and last quarter, although they are phases of lesser brightness. The first and last quarter phases occur at greatest elongation east and west of the Sun, respectively. At both of these times Mercury’s separation from the Sun ranges anywhere from 17.9° at perihelion to 27.8° at aphelion.[145][146] At greatest western elongation, Mercury rises at its earliest before sunrise, and at greatest eastern elongation, it sets at its latest after sunset.[147]
False-color image of Carnegie Rupes, a tectonic landform—high terrain (red); low (blue).
Mercury is more often and easily visible from the Southern Hemisphere than from the Northern. This is because Mercury’s maximum western elongation occurs only during early autumn in the Southern Hemisphere, whereas its greatest eastern elongation happens only during late winter in the Southern Hemisphere.[147] In both of these cases, the angle at which the planet’s orbit intersects the horizon is maximized, allowing it to rise several hours before sunrise in the former instance and not set until several hours after sundown in the latter from southern mid-latitudes, such as Argentina and South Africa.[147]
An alternate method for viewing Mercury involves observing the planet during daylight hours when conditions are clear, ideally when it is at its greatest elongation. This allows the planet to be found easily, even when using telescopes with 8 cm (3.1 in) apertures. However, great care must be taken to obstruct the Sun from sight because of the extreme risk for eye damage.[148] This method bypasses the limitation of twilight observing when the ecliptic is located at a low elevation (e.g. on autumn evenings).
Ground-based telescope observations of Mercury reveal only an illuminated partial disk with limited detail. The first of two spacecraft to visit the planet was Mariner 10, which mapped about 45% of its surface from 1974 to 1975. The second is the MESSENGER spacecraft, which after three Mercury flybys between 2008 and 2009, attained orbit around Mercury on March 17, 2011,[149] to study and map the rest of the planet.[150]
The Hubble Space Telescope cannot observe Mercury at all, due to safety procedures that prevent its pointing too close to the Sun.[151]
Because the shift of 0.15 revolutions in a year makes up a seven-year cycle (0.15 × 7 ≈ 1.0), in the seventh year Mercury follows almost exactly (earlier by 7 days) the sequence of phenomena it showed seven years before.[145]
Observation history
Ancient astronomers
Mercury, from Liber astronomiae, 1550
The earliest known recorded observations of Mercury are from the MUL.APIN tablets. These observations were most likely made by an Assyrian astronomer around the 14th century BC.[152] The cuneiform name used to designate Mercury on the MUL.APIN tablets is transcribed as UDU.IDIM.GUU4.UD («the jumping planet»).[e][153] Babylonian records of Mercury date back to the 1st millennium BC. The Babylonians called the planet Nabu after the messenger to the gods in their mythology.[154]
The Greco-Egyptian[155] astronomer Ptolemy wrote about the possibility of planetary transits across the face of the Sun in his work Planetary Hypotheses. He suggested that no transits had been observed either because planets such as Mercury were too small to see, or because the transits were too infrequent.[156]
In ancient China, Mercury was known as «the Hour Star» (Chen-xing 辰星). It was associated with the direction north and the phase of water in the Five Phases system of metaphysics.[157] Modern Chinese, Korean, Japanese and Vietnamese cultures refer to the planet literally as the «water star» (水星), based on the Five elements.[158][159][160] Hindu mythology used the name Budha for Mercury, and this god was thought to preside over Wednesday.[161] The god Odin (or Woden) of Germanic paganism was associated with the planet Mercury and Wednesday.[162] The Maya may have represented Mercury as an owl (or possibly four owls; two for the morning aspect and two for the evening) that served as a messenger to the underworld.[163]
In medieval Islamic astronomy, the Andalusian astronomer Abū Ishāq Ibrāhīm al-Zarqālī in the 11th century described the deferent of Mercury’s geocentric orbit as being oval, like an egg or a pignon, although this insight did not influence his astronomical theory or his astronomical calculations.[164][165] In the 12th century, Ibn Bajjah observed «two planets as black spots on the face of the Sun», which was later suggested as the transit of Mercury and/or Venus by the Maragha astronomer Qotb al-Din Shirazi in the 13th century.[166] (Note that most such medieval reports of transits were later taken as observations of sunspots.[167])
In India, the Kerala school astronomer Nilakantha Somayaji in the 15th century developed a partially heliocentric planetary model in which Mercury orbits the Sun, which in turn orbits Earth, similar to the Tychonic system later proposed by Tycho Brahe in the late 16th century.[168]
Ground-based telescopic research
Transit of Mercury. Mercury is visible as a black dot below and to the left of center. The dark area above the center of the solar disk is a sunspot.
Elongation is the angle between the Sun and the planet, with Earth as the reference point. Mercury appears close to the Sun.
The first telescopic observations of Mercury were made by Thomas Harriot and Galileo from 1610. In 1612, Simon Marius observed the brightness of Mercury varied with the planet’s orbital position and concluded it had phases «in the same way as Venus and the Moon».[169] In 1631, Pierre Gassendi made the first telescopic observations of the transit of a planet across the Sun when he saw a transit of Mercury predicted by Johannes Kepler. In 1639, Giovanni Zupi used a telescope to discover that the planet had orbital phases similar to Venus and the Moon. The observation demonstrated conclusively that Mercury orbited around the Sun.[32]
A rare event in astronomy is the passage of one planet in front of another (occultation), as seen from Earth. Mercury and Venus occult each other every few centuries, and the event of May 28, 1737 is the only one historically observed, having been seen by John Bevis at the Royal Greenwich Observatory.[170] The next occultation of Mercury by Venus will be on December 3, 2133.[171]
The difficulties inherent in observing Mercury mean that it was far less studied than the other planets. In 1800, Johann Schröter made observations of surface features, claiming to have observed 20-kilometre-high (12 mi) mountains. Friedrich Bessel used Schröter’s drawings to erroneously estimate the rotation period as 24 hours and an axial tilt of 70°.[172] In the 1880s, Giovanni Schiaparelli mapped the planet more accurately, and suggested that Mercury’s rotational period was 88 days, the same as its orbital period due to tidal locking.[173] This phenomenon is known as synchronous rotation. The effort to map the surface of Mercury was continued by Eugenios Antoniadi, who published a book in 1934 that included both maps and his own observations.[100] Many of the planet’s surface features, particularly the albedo features, take their names from Antoniadi’s map.[174]
In June 1962, Soviet scientists at the Institute of Radio-engineering and Electronics of the USSR Academy of Sciences, led by Vladimir Kotelnikov, became the first to bounce a radar signal off Mercury and receive it, starting radar observations of the planet.[175][176][177] Three years later, radar observations by Americans Gordon H. Pettengill and Rolf B. Dyce, using the 300-meter Arecibo radio telescope in Puerto Rico, showed conclusively that the planet’s rotational period was about 59 days.[178][179] The theory that Mercury’s rotation was synchronous had become widely held, and it was a surprise to astronomers when these radio observations were announced. If Mercury were tidally locked, its dark face would be extremely cold, but measurements of radio emission revealed that it was much hotter than expected. Astronomers were reluctant to drop the synchronous rotation theory and proposed alternative mechanisms such as powerful heat-distributing winds to explain the observations.[180]
Water ice (yellow) at Mercury’s north polar region
Italian astronomer Giuseppe Colombo noted that the rotation value was about two-thirds of Mercury’s orbital period, and proposed that the planet’s orbital and rotational periods were locked into a 3:2 rather than a 1:1 resonance.[181] Data from Mariner 10 subsequently confirmed this view.[182] This means that Schiaparelli’s and Antoniadi’s maps were not «wrong». Instead, the astronomers saw the same features during every second orbit and recorded them, but disregarded those seen in the meantime, when Mercury’s other face was toward the Sun, because the orbital geometry meant that these observations were made under poor viewing conditions.[172]
Ground-based optical observations did not shed much further light on Mercury, but radio astronomers using interferometry at microwave wavelengths, a technique that enables removal of the solar radiation, were able to discern physical and chemical characteristics of the subsurface layers to a depth of several meters.[183][184] Not until the first space probe flew past Mercury did many of its most fundamental morphological properties become known. Moreover, recent technological advances have led to improved ground-based observations. In 2000, high-resolution lucky imaging observations were conducted by the Mount Wilson Observatory 1.5 meter Hale telescope. They provided the first views that resolved surface features on the parts of Mercury that were not imaged in the Mariner 10 mission.[185] Most of the planet has been mapped by the Arecibo radar telescope, with 5 km (3.1 mi) resolution, including polar deposits in shadowed craters of what may be water ice.[186]
Research with space probes
MESSENGER being prepared for launch
Mercury transiting the Sun as viewed by the Mars rover Curiosity (June 3, 2014).[187]
Reaching Mercury from Earth poses significant technical challenges, because it orbits so much closer to the Sun than Earth. A Mercury-bound spacecraft launched from Earth must travel over 91 million kilometres (57 million miles) into the Sun’s gravitational potential well. Mercury has an orbital speed of 47.4 km/s (29.5 mi/s), whereas Earth’s orbital speed is 29.8 km/s (18.5 mi/s).[114] Therefore, the spacecraft must make a large change in velocity (delta-v) to get to Mercury and then enter orbit,[188] as compared to the delta-v required for, say, Mars planetary missions.
The potential energy liberated by moving down the Sun’s potential well becomes kinetic energy, requiring a delta-v change to do anything other than pass by Mercury. Some portion of this delta-v budget can be provided from a gravity assist during one or more fly-bys of Venus.[189] To land safely or enter a stable orbit the spacecraft would rely entirely on rocket motors. Aerobraking is ruled out because Mercury has a negligible atmosphere. A trip to Mercury requires more rocket fuel than that required to escape the Solar System completely. As a result, only three space probes have visited it so far.[190] A proposed alternative approach would use a solar sail to attain a Mercury-synchronous orbit around the Sun.[191]
Mariner 10
Mariner 10, the first probe to visit Mercury
The first spacecraft to visit Mercury was NASA’s Mariner 10 (1974–1975).[26] The spacecraft used the gravity of Venus to adjust its orbital velocity so that it could approach Mercury, making it both the first spacecraft to use this gravitational «slingshot» effect and the first NASA mission to visit multiple planets.[192] Mariner 10 provided the first close-up images of Mercury’s surface, which immediately showed its heavily cratered nature, and revealed many other types of geological features, such as the giant scarps that were later ascribed to the effect of the planet shrinking slightly as its iron core cools.[193] Unfortunately, the same face of the planet was lit at each of Mariner 10‘s close approaches. This made close observation of both sides of the planet impossible,[194] and resulted in the mapping of less than 45% of the planet’s surface.[195]
The spacecraft made three close approaches to Mercury, the closest of which took it to within 327 km (203 mi) of the surface.[196] At the first close approach, instruments detected a magnetic field, to the great surprise of planetary geologists—Mercury’s rotation was expected to be much too slow to generate a significant dynamo effect. The second close approach was primarily used for imaging, but at the third approach, extensive magnetic data were obtained. The data revealed that the planet’s magnetic field is much like Earth’s, which deflects the solar wind around the planet. For many years after the Mariner 10 encounters, the origin of Mercury’s magnetic field remained the subject of several competing theories.[197][198]
On March 24, 1975, just eight days after its final close approach, Mariner 10 ran out of fuel. Because its orbit could no longer be accurately controlled, mission controllers instructed the probe to shut down.[199] Mariner 10 is thought to be still orbiting the Sun, passing close to Mercury every few months.[200]
MESSENGER
Estimated details of the impact of MESSENGER on April 30, 2015
A second NASA mission to Mercury, named MESSENGER (MErcury Surface, Space ENvironment, GEochemistry, and Ranging), was launched on August 3, 2004. It made a fly-by of Earth in August 2005, and of Venus in October 2006 and June 2007 to place it onto the correct trajectory to reach an orbit around Mercury.[201] A first fly-by of Mercury occurred on January 14, 2008, a second on October 6, 2008,[202] and a third on September 29, 2009.[203] Most of the hemisphere not imaged by Mariner 10 was mapped during these fly-bys. The probe successfully entered an elliptical orbit around the planet on March 18, 2011. The first orbital image of Mercury was obtained on March 29, 2011. The probe finished a one-year mapping mission,[202] and then entered a one-year extended mission into 2013. In addition to continued observations and mapping of Mercury, MESSENGER observed the 2012 solar maximum.[204]
The mission was designed to clear up six key issues: Mercury’s high density, its geological history, the nature of its magnetic field, the structure of its core, whether it has ice at its poles, and where its tenuous atmosphere comes from. To this end, the probe carried imaging devices that gathered much-higher-resolution images of much more of Mercury than Mariner 10, assorted spectrometers to determine abundances of elements in the crust, and magnetometers and devices to measure velocities of charged particles. Measurements of changes in the probe’s orbital velocity were expected to be used to infer details of the planet’s interior structure.[205] MESSENGER‘s final maneuver was on April 24, 2015, and it crashed into Mercury’s surface on April 30, 2015.[206][207][208] The spacecraft’s impact with Mercury occurred near 3:26 pm EDT on April 30, 2015, leaving a crater estimated to be 16 m (52 ft) in diameter.[209]
BepiColombo
The European Space Agency and the Japanese Space Agency developed and launched a joint mission called BepiColombo, which will orbit Mercury with two probes: one to map the planet and the other to study its magnetosphere.[210] Launched on October 20, 2018, BepiColombo is expected to reach Mercury in 2025.[211] It will release a magnetometer probe into an elliptical orbit, then chemical rockets will fire to deposit the mapper probe into a circular orbit. Both probes will operate for one terrestrial year.[210] The mapper probe carries an array of spectrometers similar to those on MESSENGER, and will study the planet at many different wavelengths including infrared, ultraviolet, X-ray and gamma ray.[212] BepiColombo conducted the first of its six planned Mercury flybys on October 1, 2021.[213]
See also
- Outline of Mercury (planet)
- Budha, a deity identified with the planet in Hindu astrology
- Colonization of Mercury
- Mercury in astrology
- Mercury in fiction
Notes
- ^ In astronomy, the words «rotation» and «revolution» have different meanings. «Rotation» is the turning of a body about an axis that passes through the body, as in «Earth rotates once a day.» «Revolution» is motion around a centre that is external to the body, usually in orbit, as in «Earth takes a year for each revolution around the Sun.» The verbs «rotate» and «revolve» mean doing rotation and revolution, respectively.
- ^ Pluto was considered a planet from its discovery in 1930 to 2006, but after that it has been reclassified as a dwarf planet. Pluto’s orbital eccentricity is greater than Mercury’s. Pluto is also smaller than Mercury, but was thought to be larger until 1976.
- ^ The Sun’s total angular displacement during its apparent retrograde motion as seen from the surface of Mercury is ~1.23°, while the Sun’s angular diameter when the apparent retrograde motion begins and ends is ~1.71°, increasing to ~1.73° at perihelion (midway through the retrograde motion).
- ^ It is important to be clear about the meaning of «closeness». In the astronomical literature, the term «closest planets» often means «the two planets that approach each other most closely». In other words, the orbits of the two planets approach each other most closely. However, this does not mean that the two planets are closest over time. For example, essentially because Mercury is closer to the Sun than Venus, Mercury spends more time in proximity to Earth; it could, therefore, be said that Mercury is the planet that is «closest to Earth when averaged over time». However, using this time-average definition of ‘closeness’—as noted above—it turns out that Mercury is the closest planet to all other planets in the solar system. For that reason, arguably, the proximity-definition is not particularly helpful. An episode of the BBC Radio 4 programme «More or Less» explains the different notions of proximity well.[19]
- ^ Some sources precede the cuneiform transcription with «MUL». «MUL» is a cuneiform sign that was used in the Sumerian language to designate a star or planet, but it is not considered part of the actual name. The «4» is a reference number in the Sumero–Akkadian transliteration system to designate which of several syllables a certain cuneiform sign is most likely designating.
References
- ^ «Mercurian». Lexico UK English Dictionary. Oxford University Press. Archived from the original on March 27, 2020.
- ^ «Mercurial». Lexico UK English Dictionary UK English Dictionary. Oxford University Press. Archived from the original on December 22, 2019.
- ^ a b c d e f g h i j k l m Williams, David R. (November 25, 2020). «Mercury Fact Sheet». NASA. Retrieved April 19, 2021.
- ^ Souami, D.; Souchay, J. (July 2012). «The solar system’s invariable plane». Astronomy & Astrophysics. 543: 11. Bibcode:2012A&A…543A.133S. doi:10.1051/0004-6361/201219011. A133.
- ^ Yeomans, Donald K. (April 7, 2008). «HORIZONS Web-Interface for Mercury Major Body». JPL Horizons On-Line Ephemeris System. Retrieved April 7, 2008. – Select «Ephemeris Type: Orbital Elements», «Time Span: 2000-01-01 12:00 to 2000-01-02». («Target Body: Mercury» and «Center: Sun» should be defaulted to.) Results are instantaneous osculating values at the precise J2000 epoch.
- ^ a b c d e f g Davis, Phillips; Barnett, Amanda (February 15, 2021). «Mercury». Solar System Exploration. NASA Jet Propulsion Laboratory. Retrieved April 21, 2021.
- ^ Seidelmann, P. Kenneth; Archinal, Brent A.; A’Hearn, Michael F.; et al. (2007). «Report of the IAU/IAG Working Group on cartographic coordinates and rotational elements: 2006». Celestial Mechanics and Dynamical Astronomy. 98 (3): 155–180. Bibcode:2007CeMDA..98..155S. doi:10.1007/s10569-007-9072-y. S2CID 122772353.
- ^ Mazarico, Erwan; Genova, Antonio; Goossens, Sander; Lemoine, Frank G.; Neumann, Gregory A.; Zuber, Maria T.; Smith, David E.; Solomon, Sean C. (2014). «The gravity field, orientation, and ephemeris of Mercury from MESSENGER observations after three years in orbit» (PDF). Journal of Geophysical Research: Planets. 119 (12): 2417–2436. Bibcode:2014JGRE..119.2417M. doi:10.1002/2014JE004675. hdl:1721.1/97927. ISSN 2169-9097. S2CID 42430050.
- ^ a b c Margot, Jean-Luc; Peale, Stanton J.; Solomon, Sean C.; Hauck, Steven A.; Ghigo, Frank D.; Jurgens, Raymond F.; Yseboodt, Marie; Giorgini, Jon D.; Padovan, Sebastiano; Campbell, Donald B. (2012). «Mercury’s moment of inertia from spin and gravity data». Journal of Geophysical Research: Planets. 117 (E12): n/a. Bibcode:2012JGRE..117.0L09M. CiteSeerX 10.1.1.676.5383. doi:10.1029/2012JE004161. ISSN 0148-0227. S2CID 22408219.
- ^ «ESO». ESO. Retrieved June 3, 2021.
- ^ Mallama, Anthony (2017). «The spherical bolometric albedo for planet Mercury». arXiv:1703.02670 [astro-ph.EP].
- ^ Mallama, Anthony; Wang, Dennis; Howard, Russell A. (2002). «Photometry of Mercury from SOHO/LASCO and Earth». Icarus. 155 (2): 253–264. Bibcode:2002Icar..155..253M. doi:10.1006/icar.2001.6723.
- ^ «Atmospheres and Planetary Temperatures». American Chemical Society. July 18, 2013. Retrieved January 3, 2023.
- ^ a b c d Vasavada, Ashwin R.; Paige, David A.; Wood, Stephen E. (February 19, 1999). «Near-Surface Temperatures on Mercury and the Moon and the Stability of Polar Ice Deposits» (PDF). Icarus. 141 (2): 179–193. Bibcode:1999Icar..141..179V. doi:10.1006/icar.1999.6175. Figure 3 with the «TWO model»; Figure 5 for pole.
- ^ a b c Mallama, Anthony; Hilton, James L. (October 2018). «Computing apparent planetary magnitudes for The Astronomical Almanac». Astronomy and Computing. 25: 10–24. arXiv:1808.01973. Bibcode:2018A&C….25…10M. doi:10.1016/j.ascom.2018.08.002. S2CID 69912809.
- ^ a b Milillo, A.; Wurz, P.; Orsini, S.; Delcourt, D.; Kallio, E.; Killen, R. M.; Lammer, H.; Massetti, S.; Mura, A.; Barabash, S.; Cremonese, G.; Daglis, I. A.; Angelis, E.; Lellis, A. M.; Livi, S.; Mangano, V.; Torkar, K. (April 2005). «Surface-Exosphere-Magnetosphere System Of Mercury». Space Science Reviews. 117 (3–4): 397–443. Bibcode:2005SSRv..117..397M. doi:10.1007/s11214-005-3593-z. S2CID 122285073.
- ^ a b Berezhnoy, Alexey A. (January 2018). «Chemistry of impact events on Mercury». Icarus. 300: 210–222. Bibcode:2018Icar..300..210B. doi:10.1016/j.icarus.2017.08.034.
- ^ a b «Venus is not Earth’s closest neighbor». Physics Today. AIP Publishing. March 12, 2019. doi:10.1063/pt.6.3.20190312a. ISSN 1945-0699. S2CID 241077611.
- ^ a b c Harford, Tim (January 11, 2019). «BBC Radio 4 – More or Less, Sugar, Outdoors Play and Planets». BBC.
Oliver Hawkins, more or less alumnus and statistical legend, wrote some code for us, which calculated which planet was closest to the Earth on each day for the past 50 years, and then sent the results to David A. Rothery, professor of planetary geosciences at the Open University.
- ^ Elkins-Tanton, Linda T. (2006). Uranus, Neptune, Pluto, and the Outer Solar System. Infobase Publishing. p. 51. ISBN 978-1-4381-0729-5. Extract of page 51
- ^ «Animated clip of orbit and rotation of Mercury». Sciencenetlinks.com.
- ^ a b Prockter, Louise (2005). Ice in the Solar System (PDF). Vol. 26. Johns Hopkins APL Technical Digest. Retrieved July 27, 2009.
- ^ «NASA Completes MESSENGER Mission with Expected Impact on Mercury’s Surface». Archived from the original on May 3, 2015. Retrieved April 30, 2015.
- ^ «From Mercury orbit, MESSENGER watches a lunar eclipse». Planetary Society. October 10, 2014. Retrieved January 23, 2015.
- ^ «Innovative use of pressurant extends MESSENGER’s Mercury mission». Astronomy.com. December 29, 2014. Retrieved January 22, 2015.
- ^ a b c Dunne, James A.; Burgess, Eric (1978). «Chapter One». The Voyage of Mariner 10 – Mission to Venus and Mercury. NASA History Office.
- ^ Στίλβων, Ἑρμῆς. Liddell, Henry George; Scott, Robert; A Greek–English Lexicon at the Perseus Project.
- ^ «Greek Names of the Planets». April 25, 2010. Retrieved July 14, 2012.
Ermis is the Greek name of the planet Mercury, which is the closest planet to the Sun. It is named after the Greek God of commerce, Ermis or Hermes, who was also the messenger of the Ancient Greek gods.
See also the Greek article about the planet. - ^ Antoniadi, Eugène Michel (1974). The Planet Mercury. Translated from French by Moore, Patrick. Shaldon, Devon: Keith Reid Ltd. pp. 9–11. ISBN 978-0-904094-02-2.
- ^ Duncan, John Charles (1946). Astronomy: A Textbook. Harper & Brothers. p. 125.
The symbol for Mercury represents the Caduceus, a wand with two serpents twined around it, which was carried by the messenger of the gods.
- ^
Jones, Alexander (1999). Astronomical papyri from Oxyrhynchus. pp. 62–63. ISBN 9780871692337.It is now possible to trace the medieval symbols for at least four of the five planets to forms that occur in some of the latest papyrus horoscopes ([ P.Oxy. ] 4272, 4274, 4275 […]). Mercury’s is a stylized caduceus.
- ^ a b c d e f g h Strom, Robert G.; Sprague, Ann L. (2003). Exploring Mercury: the iron planet. Springer. ISBN 978-1-85233-731-5.
- ^ Talbert, Tricia, ed. (March 21, 2012). «MESSENGER Provides New Look at Mercury’s Surprising Core and Landscape Curiosities». NASA.
- ^ «Scientists find evidence Mercury has a solid inner core». AGU Newsroom. Retrieved April 17, 2019.
- ^ «Mercury». US Geological Survey. May 8, 2003. Archived from the original on September 29, 2006. Retrieved November 26, 2006.
- ^ Lyttleton, Raymond A. (1969). «On the Internal Structures of Mercury and Venus». Astrophysics and Space Science. 5 (1): 18–35. Bibcode:1969Ap&SS…5…18L. doi:10.1007/BF00653933. S2CID 122572625.
- ^ Hauck, Steven A.; Margot, Jean-Luc; Solomon, Sean C.; Phillips, Roger J.; Johnson, Catherine L.; Lemoine, Frank G.; Mazarico, Erwan; McCoy, Timothy J.; Padovan, Sebastiano; Peale, Stanton J.; Perry, Mark E.; Smith, David E.; Zuber, Maria T. (2013). «The curious case of Mercury’s internal structure». Journal of Geophysical Research: Planets. 118 (6): 1204–1220. Bibcode:2013JGRE..118.1204H. doi:10.1002/jgre.20091. hdl:1721.1/85633. S2CID 17668886.
- ^ Gold, Lauren (May 3, 2007). «Mercury has molten core, Cornell researcher shows». Chronicle. Cornell University. Retrieved May 12, 2008.
- ^ Finley, Dave (May 3, 2007). «Mercury’s Core Molten, Radar Study Shows». National Radio Astronomy Observatory. Retrieved May 12, 2008.
- ^ a b Spohn, Tilman; Sohl, Frank; Wieczerkowski, Karin; Conzelmann, Vera (2001). «The interior structure of Mercury: what we know, what we expect from BepiColombo». Planetary and Space Science. 49 (14–15): 1561–1570. Bibcode:2001P&SS…49.1561S. doi:10.1016/S0032-0633(01)00093-9.
- ^ Gallant, Roy A. (1986). The National Geographic Picture Atlas of Our Universe (2nd ed.). National Geographic Society. ISBN 9780870446443.
- ^ Padovan, Sebastiano; Wieczorek, Mark A.; Margot, Jean-Luc; Tosi, Nicola; Solomon, Sean C. (2015). «Thickness of the crust of Mercury from geoid-to-topography ratios». Geophysical Research Letters. 42 (4): 1029. Bibcode:2015GeoRL..42.1029P. doi:10.1002/2014GL062487. S2CID 31442257.
- ^ Solomon, Sean C.; Nittler, Larry R.; Anderson, Brian J. (December 20, 2018). Mercury: The View after MESSENGER. Cambridge University Press. p. 534. ISBN 978-1-107-15445-2.
- ^ Sori, Michael M. (May 2018). «A thin, dense crust for Mercury». Earth and Planetary Science Letters. 489: 92–99. Bibcode:2018E&PSL.489…92S. doi:10.1016/j.epsl.2018.02.033.
- ^ Schenk, Paul M.; Melosh, H. Jay (March 1994). «Lobate Thrust Scarps and the Thickness of Mercury’s Lithosphere». Abstracts of the 25th Lunar and Planetary Science Conference. 1994: 1994LPI….25.1203S. Bibcode:1994LPI….25.1203S.
- ^ Watters, T. R.; Nimmo, F.; Robinson, M. S. (2004). Chronology of Lobate Scarp Thrust Faults and the Mechanical Structure of Mercury’s Lithosphere. Lunar and Planetary Science Conference. p. 1886. Bibcode:2004LPI….35.1886W.
- ^ Watters, Thomas R.; Robinson, Mark S.; Cook, Anthony C. (November 1998). «Topography of lobate scarps on Mercury; new constraints on the planet’s contraction». Geology. 26 (11): 991–994. Bibcode:1998Geo….26..991W. doi:10.1130/0091-7613(1998)026<0991:TOLSOM>2.3.CO;2.
- ^ a b c d Benz, W.; Slattery, W. L.; Cameron, Alastair G. W. (1988). «Collisional stripping of Mercury’s mantle». Icarus. 74 (3): 516–528. Bibcode:1988Icar…74..516B. doi:10.1016/0019-1035(88)90118-2.
- ^ a b Cameron, Alastair G. W. (1985). «The partial volatilization of Mercury». Icarus. 64 (2): 285–294. Bibcode:1985Icar…64..285C. doi:10.1016/0019-1035(85)90091-0.
- ^ Weidenschilling, Stuart J. (1987). «Iron/silicate fractionation and the origin of Mercury». Icarus. 35 (1): 99–111. Bibcode:1978Icar…35…99W. doi:10.1016/0019-1035(78)90064-7.
- ^ Sappenfield, Mark (September 29, 2011). «Messenger’s message from Mercury: Time to rewrite the textbooks». The Christian Science Monitor. Retrieved August 21, 2017.
- ^ «BepiColombo». Science & Technology. European Space Agency. Retrieved April 7, 2008.
- ^ Cartwright, Jon (September 30, 2011). «Messenger sheds light on Mercury’s formation». Chemistry World. Retrieved August 21, 2017.
- ^ Morris, Jefferson (November 10, 2008). «Laser Altimetry». Aviation Week & Space Technology. 169 (18): 18.
Mercury’s crust is more analogous to a marbled cake than a layered cake.
- ^ Hughes, E. T.; Vaughan, W. M. (March 2012). Albedo Features of Mercury. 43rd Lunar and Planetary Science Conference, held March 19–23, 2012 at The Woodlands, Texas. Vol. 1659. Bibcode:2012LPI….43.2151H. 2151.
- ^ Blue, Jennifer (April 11, 2008). «Gazetteer of Planetary Nomenclature». US Geological Survey. Retrieved April 11, 2008.
- ^ a b Dunne, James A.; Burgess, Eric (1978). «Chapter Seven». The Voyage of Mariner 10 – Mission to Venus and Mercury. NASA History Office. Retrieved May 28, 2008.
- ^ Nittler, Larry R.; Weider, Shoshana Z. (2019). «The Surface Composition of Mercury». Elements. 15 (1): 33–38. doi:10.2138/gselements.15.1.33. S2CID 135051680.
- ^ «Categories for Naming Features on Planets and Satellites». US Geological Survey. Retrieved August 20, 2011.
- ^ Strom, Robert G. (1979). «Mercury: a post-Mariner assessment». Space Science Reviews. 24 (1): 3–70. Bibcode:1979SSRv…24….3S. doi:10.1007/BF00221842. S2CID 122563809.
- ^ Broadfoot, A. Lyle; Kumar, Shailendra; Belton, Michael J. S.; McElroy, Michael B. (July 12, 1974). «Mercury’s Atmosphere from Mariner 10: Preliminary Results». Science. 185 (4146): 166–169. Bibcode:1974Sci…185..166B. doi:10.1126/science.185.4146.166. PMID 17810510. S2CID 7790470.
- ^ Geology of the solar system. IMAP 2596. U.S. Geological Survey. 1997. doi:10.3133/i2596.
- ^ Head, James W.; Solomon, Sean C. (1981). «Tectonic Evolution of the Terrestrial Planets» (PDF). Science. 213 (4503): 62–76. Bibcode:1981Sci…213…62H. CiteSeerX 10.1.1.715.4402. doi:10.1126/science.213.4503.62. hdl:2060/20020090713. PMID 17741171. Archived from the original (PDF) on July 21, 2018. Retrieved October 25, 2017.
- ^ «Scientists see Mercury in a new light». Science Daily. February 28, 2008. Retrieved April 7, 2008.
- ^ a b c d e f g Spudis, Paul D. (2001). «The Geological History of Mercury». Workshop on Mercury: Space Environment, Surface, and Interior, Chicago (1097): 100. Bibcode:2001mses.conf..100S.
- ^ Ritzel, Rebecca (December 20, 2012). «Ballet isn’t rocket science, but the two aren’t mutually exclusive, either». The Washington Post. Washington, D.C., United States. Retrieved December 22, 2012.
- ^ Shiga, David (January 30, 2008). «Bizarre spider scar found on Mercury’s surface». NewScientist.com news service.
- ^ Schultz, Peter H.; Gault, Donald E. (1975). «Seismic effects from major basin formations on the moon and Mercury». Earth, Moon, and Planets. 12 (2): 159–175. Bibcode:1975Moon…12..159S. doi:10.1007/BF00577875. S2CID 121225801.
- ^ Wieczorek, Mark A.; Zuber, Maria T. (2001). «A Serenitatis origin for the Imbrian grooves and South Pole-Aitken thorium anomaly». Journal of Geophysical Research. 106 (E11): 27853–27864. Bibcode:2001JGR…10627853W. doi:10.1029/2000JE001384. Retrieved May 12, 2008.
- ^ Fassett, Caleb I.; Head, James W.; Baker, David M. H.; Zuber, Maria T.; Smith, David E.; Neumann, Gregory A.; Solomon, Sean C.; Klimczak, Christian; Strom, Robert G.; Chapman, Clark R.; Prockter, Louise M.; Phillips, Roger J.; Oberst, Jürgen; Preusker, Frank (October 2012). «Large impact basins on Mercury: Global distribution, characteristics, and modification history from MESSENGER orbital data». Journal of Geophysical Research. 117. 15 pp. Bibcode:2012JGRE..117.0L08F. doi:10.1029/2012JE004154. E00L08.
- ^ Denevi, Brett W.; Robinson, Mark S. (2008). «Albedo of Immature Mercurian Crustal Materials: Evidence for the Presence of Ferrous Iron». Lunar and Planetary Science. 39 (1391): 1750. Bibcode:2008LPI….39.1750D.
- ^ a b Wagner, Roland J.; Wolf, Ursula; Ivanov, Boris A.; Neukum, Gerhard (October 4–5, 2001). Application of an Updated Impact Cratering Chronology Model to Mercury’ s Time-Stratigraphic System. Workshop on Mercury: Space Environment, Surface, and Interior. Proceedings of a workshop held at The Field Museum. Chicago, IL: Lunar and Planetary Science Institute. p. 106. Bibcode:2001mses.conf..106W.
- ^ Schleicher, Lisa S.; Watters, Thomas R.; Martin, Aaron J.; Banks, Maria E. (October 2019). «Wrinkle ridges on Mercury and the Moon within and outside of mascons». Icarus. 331: 226–237. Bibcode:2019Icar..331..226S. doi:10.1016/j.icarus.2019.04.013. S2CID 150072193.
- ^ a b Choi, Charles Q. (September 26, 2016). «Mercuryquakes May Currently Shake Up the Tiny Planet». Space.com. Retrieved September 28, 2016.
- ^ Dzurisin, Daniel (October 10, 1978). «The tectonic and volcanic history of Mercury as inferred from studies of scarps, ridges, troughs, and other lineaments». Journal of Geophysical Research. 83 (B10): 4883–4906. Bibcode:1978JGR….83.4883D. doi:10.1029/JB083iB10p04883.
- ^ a b Watters, Thomas R.; Daud, Katie; Banks, Maria E.; Selvans, Michelle M.; Chapman, Clark R.; Ernst, Carolyn M. (September 26, 2016). «Recent tectonic activity on Mercury revealed by small thrust fault scarps». Nature Geoscience. 9 (10): 743–747. Bibcode:2016NatGe…9..743W. doi:10.1038/ngeo2814.
- ^ Giacomini, L.; Massironi, M.; Galluzzi, V.; Ferrari, S.; Palumbo, P. (May 2020). «Dating long thrust systems on Mercury: New clues on the thermal evolution of the planet». Geoscience Frontiers. 11 (3): 855–870. doi:10.1016/j.gsf.2019.09.005. S2CID 210298205.
- ^ Kerber, Laura; Head, James W.; Solomon, Sean C.; Murchie, Scott L.; Blewett, David T. (August 15, 2009). «Explosive volcanic eruptions on Mercury: Eruption conditions, magma volatile content, and implications for interior volatile abundances». Earth and Planetary Science Letters. 285 (3–4): 263–271. Bibcode:2009E&PSL.285..263K. doi:10.1016/j.epsl.2009.04.037.
- ^ Head, James W.; Chapman, Clark R.; Strom, Robert G.; Fassett, Caleb I.; Denevi, Brett W. (September 30, 2011). «Flood Volcanism in the Northern High Latitudes of Mercury Revealed by MESSENGER» (PDF). Science. 333 (6051): 1853–1856. Bibcode:2011Sci…333.1853H. doi:10.1126/science.1211997. PMID 21960625. S2CID 7651992.
- ^ Thomas, Rebecca J.; Rothery, David A.; Conway, Susan J.; Anand, Mahesh (September 16, 2014). «Long-lived explosive volcanism on Mercury». Geophysical Research Letters. 41 (17): 6084–6092. Bibcode:2014GeoRL..41.6084T. doi:10.1002/2014GL061224. S2CID 54683272.
- ^ a b c Groudge, Timothy A.; Head, James W. (March 2014). «Global inventory and characterization of pyroclastic deposits on Mercury: New insights into pyroclastic activity from MESSENGER orbital data» (PDF). Journal of Geophysical Research. 119 (3): 635–658. Bibcode:2014JGRE..119..635G. doi:10.1002/2013JE004480. S2CID 14393394.
- ^ a b c Rothery, David A.; Thomas, Rebeca J.; Kerber, Laura (January 1, 2014). «Prolonged eruptive history of a compound volcano on Mercury: Volcanic and tectonic implications» (PDF). Earth and Planetary Science Letters. 385: 59–67. Bibcode:2014E&PSL.385…59R. doi:10.1016/j.epsl.2013.10.023.
- ^ a b Chang, Kenneth (November 29, 2012). «On Closest Planet to the Sun, NASA Finds Lots of Ice». The New York Times. p. A3. Archived from the original on November 29, 2012.
Sean C. Solomon, the principal investigator for MESSENGER, said there was enough ice there to encase Washington, D.C., in a frozen block two and a half miles deep.
- ^ Lewis, John S. (2004). Physics and Chemistry of the Solar System (2nd ed.). Academic Press. p. 463. ISBN 978-0-12-446744-6.
- ^ Murdock, Thomas L.; Ney, Edward P. (1970). «Mercury: The Dark-Side Temperature». Science. 170 (3957): 535–537. Bibcode:1970Sci…170..535M. doi:10.1126/science.170.3957.535. PMID 17799708. S2CID 38824994.
- ^ Lewis, John S. (2004). Physics and Chemistry of the Solar System. Academic Press. ISBN 978-0-12-446744-6. Retrieved June 3, 2008.
- ^ Ingersoll, Andrew P.; Svitek, Tomas; Murray, Bruce C. (1992). «Stability of polar frosts in spherical bowl-shaped craters on the Moon, Mercury, and Mars». Icarus. 100 (1): 40–47. Bibcode:1992Icar..100…40I. doi:10.1016/0019-1035(92)90016-Z.
- ^ Slade, Martin A.; Butler, Bryan J.; Muhleman, Duane O. (1992). «Mercury radar imaging – Evidence for polar ice». Science. 258 (5082): 635–640. Bibcode:1992Sci…258..635S. doi:10.1126/science.258.5082.635. PMID 17748898. S2CID 34009087.
- ^ Williams, David R. (June 2, 2005). «Ice on Mercury». NASA Goddard Space Flight Center. Retrieved May 23, 2008.
- ^ a b c Rawlins, Katherine; Moses, Julianne I.; Zahnle, Kevin J. (1995). «Exogenic Sources of Water for Mercury’s Polar Ice». Bulletin of the American Astronomical Society. 27: 1117. Bibcode:1995DPS….27.2112R.
- ^ Harmon, John K.; Perillat, Phil J.; Slade, Martin A. (2001). «High-Resolution Radar Imaging of Mercury’s North Pole». Icarus. 149 (1): 1–15. Bibcode:2001Icar..149….1H. doi:10.1006/icar.2000.6544.
- ^ Domingue DL, Koehn PL, et al. (2009). «Mercury’s Atmosphere: A Surface-Bounded Exosphere». Space Science Reviews. 131 (1–4): 161–186. Bibcode:2007SSRv..131..161D. doi:10.1007/s11214-007-9260-9. S2CID 121301247.
- ^ Hunten, Donald M.; Shemansky, Donald Eugene; Morgan, Thomas Hunt (1988). «The Mercury atmosphere». In Vilas, Faith; Chapman, Clark R.; Shapley Matthews, Mildred (eds.). Mercury. University of Arizona Press. ISBN 978-0-8165-1085-6.
- ^ Lakdawalla, Emily (July 3, 2008). «MESSENGER Scientists «Astonished» to Find Water in Mercury’s Thin Atmosphere». The Planetary Society. Retrieved May 18, 2009.
- ^ Zurbuchen TH, Raines JM, et al. (2008). «MESSENGER Observations of the Composition of Mercury’s Ionized Exosphere and Plasma Environment». Science. 321 (5885): 90–92. Bibcode:2008Sci…321…90Z. doi:10.1126/science.1159314. PMID 18599777. S2CID 206513512.
- ^ «Instrument Shows What Planet Mercury Is Made Of». University of Michigan. June 30, 2008. Retrieved May 18, 2009.
- ^ Killen, Rosemary; Cremonese, Gabrielle; et al. (2007). «Processes that Promote and Deplete the Exosphere of Mercury». Space Science Reviews. 132 (2–4): 433–509. Bibcode:2007SSRv..132..433K. doi:10.1007/s11214-007-9232-0. S2CID 121944553.
- ^ Killen, Rosemary M.; Hahn, Joseph M. (December 10, 2014). «Impact Vaporization as a Possible Source of Mercury’s Calcium Exosphere». Icarus. 250: 230–237. Bibcode:2015Icar..250..230K. doi:10.1016/j.icarus.2014.11.035. hdl:2060/20150010116.
- ^ McClintock, William E.; Vervack, Ronald J.; et al. (2009). «MESSENGER Observations of Mercury’s Exosphere: Detection of Magnesium and Distribution of Constituents». Science. 324 (5927): 610–613. Bibcode:2009Sci…324..610M. doi:10.1126/science.1172525. PMID 19407195. S2CID 5578520.
- ^ a b c d e Beatty, J. Kelly; Petersen, Carolyn Collins; Chaikin, Andrew (1999). The New Solar System. Cambridge University Press. ISBN 978-0-521-64587-4.
- ^ «Mercury». NASA. October 19, 2021. Retrieved July 4, 2022.
- ^ Hall, Shannon (March 24, 2020). «Life on the Planet Mercury? ‘It’s Not Completely Nuts’ – A new explanation for the rocky world’s jumbled landscape opens a possibility that it could have had ingredients for habitability». The New York Times. Archived from the original on March 24, 2020. Retrieved March 26, 2020.
- ^ Rodriguez, J. Alexis P.; Leonard, Gregory J.; Kargel, Jeffrey S.; Domingue, Deborah; Berman, Daniel C.; Banks, Maria; Zarroca, Mario; Linares, Rogelio; Marchi, Simone; Baker, Victor R.; Webster, Kevin D.; Sykes, Mark (March 16, 2020). «The Chaotic Terrains of Mercury Reveal a History of Planetary Volatile Retention and Loss in the Innermost Solar System». Scientific Reports. 10 (4737): 4737. Bibcode:2020NatSR..10.4737R. doi:10.1038/s41598-020-59885-5. PMC 7075900. PMID 32179758.
- ^ «Vast Collapsed Terrains on Mercury Might be Windows Into Ancient – Possibly Habitable – Volatile-Rich Materials». Planetary Science Institute. March 16, 2020. Retrieved August 27, 2022.
- ^ Seeds, Michael A. (2004). Astronomy: The Solar System and Beyond (4th ed.). Brooks Cole. ISBN 978-0-534-42111-3.
- ^ Williams, David R. (January 6, 2005). «Planetary Fact Sheets». NASA National Space Science Data Center. Retrieved August 10, 2006.
- ^ a b c «Mercury’s Internal Magnetic Field». NASA. January 30, 2008. Retrieved April 21, 2021.
- ^ Gold, Lauren (May 3, 2007). «Mercury has molten core, Cornell researcher shows». Cornell University. Retrieved April 7, 2008.
- ^ Christensen, Ulrich R. (2006). «A deep dynamo generating Mercury’s magnetic field». Nature. 444 (7122): 1056–1058. Bibcode:2006Natur.444.1056C. doi:10.1038/nature05342. PMID 17183319. S2CID 4342216.
- ^ Padovan, Sebastiano; Margot, Jean-Luc; Hauck, Steven A.; Moore, William B.; Solomon, Sean C. (April 2014). «The tides of Mercury and possible implications for its interior structure». Journal of Geophysical Research: Planets. 119 (4): 850–866. Bibcode:2014JGRE..119..850P. doi:10.1002/2013JE004459. S2CID 56282397.
- ^ a b Steigerwald, Bill (June 2, 2009). «Magnetic Tornadoes Could Liberate Mercury’s Tenuous Atmosphere». NASA Goddard Space Flight Center. Retrieved July 18, 2009.
- ^ Van Hoolst, Tim; Jacobs, Carla (2003). «Mercury’s tides and interior structure». Journal of Geophysical Research. 108 (E11): 7. Bibcode:2003JGRE..108.5121V. doi:10.1029/2003JE002126.
- ^ «Space Topics: Compare the Planets: Mercury, Venus, Earth, The Moon, and Mars». Planetary Society. Archived from the original on July 28, 2011. Retrieved April 12, 2007.
- ^ a b Williams, David R. (October 21, 2019). «Planetary Fact Sheet – Metric». NASA. Retrieved April 20, 2021.
- ^ Espenak, Fred (April 21, 2005). «Transits of Mercury». NASA/Goddard Space Flight Center. Retrieved May 20, 2008.
- ^ Biswas, Sukumar (2000). Cosmic Perspectives in Space Physics. Astrophysics and Space Science Library. Springer. p. 176. ISBN 978-0-7923-5813-8.
- ^ a b Margot, J. L.; Peale, S. J.; Jurgens, R. F.; Slade, M. A.; et al. (2007). «Large Longitude Libration of Mercury Reveals a Molten Core». Science. 316 (5825): 710–714. Bibcode:2007Sci…316..710M. doi:10.1126/science.1140514. PMID 17478713. S2CID 8863681.
- ^ Popular Astronomy: A Review of Astronomy and Allied Sciences. Goodsell Observatory of Carleton College. 1896.
although in the case of Venus the libration in longitude due to the eccentricity of the orbit amounts to only 47′ on either side of the mean position, in the case of Mercury it amounts to 23° 39′
- ^ Seligman, C. «The Rotation of Mercury». cseligman.com. NASA Flash animation. Retrieved July 31, 2019.
- ^ van Hemerlrijck, E. (August 1983). «On the Variations in the Insolation at Mercury Resulting from Oscillations of the Orbital Eccentricity». The Moon and the Planets. 29 (1): 83–93. Bibcode:1983M&P….29…83V. doi:10.1007/BF00928377. S2CID 122761699.
- ^ Mercury Closest Approaches to Earth generated with:
1. Solex 10 Archived December 20, 2008, at the Wayback Machine (Text Output file Archived March 9, 2012, at the Wayback Machine)
2. Gravity Simulator charts Archived September 12, 2014, at the Wayback Machine
3. JPL Horizons 1950–2200 Archived November 6, 2015, at the Wayback Machine(3 sources are provided to address original research concerns and to support general long-term trends)
- ^ Stockman, Tom; Monroe, Gabriel; Cordner, Samuel (March 12, 2019). «Venus is not Earth’s closest neighbor». Physics Today. doi:10.1063/PT.6.3.20190312a. S2CID 241077611.
- ^ Stockman, Tom (March 7, 2019). Mercury is the closest planet to all seven other planets (video). Archived from the original on October 28, 2021. Retrieved May 29, 2019 – via YouTube.
- ^ 🌍 Which Planet is the Closest?, archived from the original on October 28, 2021, retrieved July 22, 2021
- ^ Davies, M. E. (June 10, 1975). «Surface Coordinates and Cartography of Mercury». Journal of Geophysical Research. 80 (B17): 2417–2430. Bibcode:1975JGR….80.2417D. doi:10.1029/JB080i017p02417.
- ^ Davies, M. E.; Dwornik, S. E.; Gault, D. E.; Strom, R. G. (1978). NASA Atlas of Mercury. NASA Scientific and Technical Information Office.
- ^ «USGS Astrogeology: Rotation and pole position for the Sun and planets (IAU WGCCRE)». Archived from the original on October 24, 2011. Retrieved October 22, 2009.
- ^ Archinal, Brent A.; A’Hearn, Michael F.; Bowell, Edward L.; Conrad, Albert R.; et al. (2010). «Report of the IAU Working Group on Cartographic Coordinates and Rotational Elements: 2009». Celestial Mechanics and Dynamical Astronomy. 109 (2): 101–135. Bibcode:2011CeMDA.109..101A. doi:10.1007/s10569-010-9320-4. ISSN 0923-2958. S2CID 189842666.
- ^ Liu, Han-Shou; O’Keefe, John A. (1965). «Theory of Rotation for the Planet Mercury». Science. 150 (3704): 1717. Bibcode:1965Sci…150.1717L. doi:10.1126/science.150.3704.1717. PMID 17768871. S2CID 45608770.
- ^ a b Colombo, Giuseppe; Shapiro, Irwin I. (1966). «The rotation of the planet Mercury». Astrophysical Journal. 145: 296. Bibcode:1966ApJ…145..296C. doi:10.1086/148762.
- ^ Correia, Alexandre C. M.; Laskar, Jacques (2009). «Mercury’s capture into the 3/2 spin-orbit resonance including the effect of core–mantle friction». Icarus. 201 (1): 1–11. arXiv:0901.1843. Bibcode:2009Icar..201….1C. doi:10.1016/j.icarus.2008.12.034. S2CID 14778204.
- ^ Correia, Alexandre C. M.; Laskar, Jacques (2004). «Mercury’s capture into the 3/2 spin-orbit resonance as a result of its chaotic dynamics». Nature. 429 (6994): 848–850. Bibcode:2004Natur.429..848C. doi:10.1038/nature02609. PMID 15215857. S2CID 9289925.
- ^ Noyelles, B.; Frouard, J.; Makarov, V. V. & Efroimsky, M. (2014). «Spin-orbit evolution of Mercury revisited». Icarus. 241 (2014): 26–44. arXiv:1307.0136. Bibcode:2014Icar..241…26N. doi:10.1016/j.icarus.2014.05.045. S2CID 53690707.
- ^ Laskar, Jacques (March 18, 2008). «Chaotic diffusion in the Solar System». Icarus. 196 (1): 1–15. arXiv:0802.3371. Bibcode:2008Icar..196….1L. doi:10.1016/j.icarus.2008.02.017. S2CID 11586168.
- ^ Laskar, Jacques; Gastineau, Mickaël (June 11, 2009). «Existence of collisional trajectories of Mercury, Mars and Venus with the Earth». Nature. 459 (7248): 817–819. Bibcode:2009Natur.459..817L. doi:10.1038/nature08096. PMID 19516336. S2CID 4416436.
- ^ Le Verrier, Urbain (1859). «Lettre de M. Le Verrier à M. Faye sur la théorie de Mercure et sur le mouvement du périhélie de cette planète». Comptes rendus hebdomadaires des séances de l’Académie des sciences (in French). Paris. 49: 379–383. (At p. 383 in the same volume Le Verrier’s report is followed by another, from Faye, enthusiastically recommending to astronomers to search for a previously undetected intra-mercurial object.)
- ^ Baum, Richard; Sheehan, William (1997). In Search of Planet Vulcan, The Ghost in Newton’s Clockwork Machine. New York: Plenum Press. ISBN 978-0-306-45567-4.
- ^ a b Clemence, Gerald M. (1947). «The Relativity Effect in Planetary Motions». Reviews of Modern Physics. 19 (4): 361–364. Bibcode:1947RvMP…19..361C. doi:10.1103/RevModPhys.19.361.
- ^ Gilvarry, John J. (1953). «Relativity Precession of the Asteroid Icarus». Physical Review. 89 (5): 1046. Bibcode:1953PhRv…89.1046G. doi:10.1103/PhysRev.89.1046.
- ^ Brown, Kevin. «6.2 Anomalous Precession». Reflections on Relativity. MathPages. Retrieved May 22, 2008.
- ^ a b Menzel, Donald H. (1964). A Field Guide to the Stars and Planets. The Peterson Field Guide Series. Boston: Houghton Mifflin Co. pp. 292–293.
- ^ Tezel, Tunç (January 22, 2003). «Total Solar Eclipse of 2006 March 29». Department of Physics at Fizik Bolumu in Turkey. Retrieved May 24, 2008.
- ^ a b Mallama, Anthony (2011). «Planetary magnitudes». Sky and Telescope. 121 (1): 51–56.
- ^ Espenak, Fred (1996). «NASA Reference Publication 1349; Venus: Twelve year planetary ephemeris, 1995–2006». Twelve Year Planetary Ephemeris Directory. NASA. Retrieved May 24, 2008.
- ^ a b Walker, John. «Mercury Chaser’s Calculator». Fourmilab Switzerland. Retrieved May 29, 2008. (look at 1964 and 2013)
- ^ «Mercury Elongation and Distance». Archived from the original on May 11, 2013. Retrieved May 30, 2008. – Numbers generated using the Solar System Dynamics Group, Horizons On-Line Ephemeris System
- ^ a b c Kelly, Patrick, ed. (2007). Observer’s Handbook 2007. Royal Astronomical Society of Canada. ISBN 978-0-9738109-3-6.
- ^ Curtis, A. C. (October 1972). «Finding Venus or Mercury in daylight». Journal of the British Astronomical Association. 82: 438–439. Bibcode:1972JBAA…82..438C.
- ^ Alers, Paul E. (March 17, 2011). «Celebrating Mercury Orbit». NASA Multimedia. Retrieved March 18, 2011.
- ^ «NASA spacecraft now circling Mercury – a first». NBC News. March 17, 2011. Retrieved March 24, 2011.
- ^ Baumgardner, Jeffrey; Mendillo, Michael; Wilson, Jody K. (2000). «A Digital High-Definition Imaging System for Spectral Studies of Extended Planetary Atmospheres. I. Initial Results in White Light Showing Features on the Hemisphere of Mercury Unimaged by Mariner 10». The Astronomical Journal. 119 (5): 2458–2464. Bibcode:2000AJ….119.2458B. doi:10.1086/301323. S2CID 17361371.
- ^ Schaefer, Bradley E. (2007). «The Latitude and Epoch for the Origin of the Astronomical Lore in MUL.APIN». American Astronomical Society Meeting 210, #42.05. 38: 157. Bibcode:2007AAS…210.4205S.
- ^ Hunger, Hermann; Pingree, David (1989). «MUL.APIN: An Astronomical Compendium in Cuneiform». Archiv für Orientforschung. 24: 146.
- ^ «MESSENGER: Mercury and Ancient Cultures». NASA JPL. 2008. Retrieved April 7, 2008.
- ^ Heath, Sir Thomas (1921). A History of Greek Mathematics. Vol. II. Oxford: Clarendon Press. pp. vii, 273.
- ^ Goldstein, Bernard R. (1996). «The Pre-telescopic Treatment of the Phases and Apparent Size of Venus». Journal for the History of Astronomy. 27: 1. Bibcode:1996JHA….27….1G. doi:10.1177/002182869602700101. S2CID 117218196.
- ^ Kelley, David H.; Milone, E. F.; Aveni, Anthony F. (2004). Exploring Ancient Skies: An Encyclopedic Survey of Archaeoastronomy. Birkhäuser. ISBN 978-0-387-95310-6.
- ^ De Groot, Jan Jakob Maria (1912). Religion in China: universism. a key to the study of Taoism and Confucianism. American lectures on the history of religions. Vol. 10. G. P. Putnam’s Sons. p. 300. Retrieved January 8, 2010.
- ^ Crump, Thomas (1992). The Japanese numbers game: the use and understanding of numbers in modern Japan. Nissan Institute/Routledge Japanese studies series. Routledge. pp. 39–40. ISBN 978-0-415-05609-0.
- ^ Hulbert, Homer Bezaleel (1909). The passing of Korea. Doubleday, Page & company. p. 426. Retrieved January 8, 2010.
- ^ Pujari, R. M.; Kolhe, Pradeep; Kumar, N. R. (2006). Pride of India: A Glimpse Into India’s Scientific Heritage. Samskrita Bharati. ISBN 978-81-87276-27-2.
- ^ Bakich, Michael E. (2000). The Cambridge Planetary Handbook. Cambridge University Press. ISBN 978-0-521-63280-5.
- ^ Milbrath, Susan (1999). Star Gods of the Maya: Astronomy in Art, Folklore and Calendars. University of Texas Press. ISBN 978-0-292-75226-9.
- ^ Samsó, Julio; Mielgo, Honorino (1994). «Ibn al-Zarqālluh on Mercury». Journal for the History of Astronomy. 25 (4): 289–96 [292]. Bibcode:1994JHA….25..289S. doi:10.1177/002182869402500403. S2CID 118108131.
- ^ Hartner, Willy (1955). «The Mercury Horoscope of Marcantonio Michiel of Venice». Vistas in Astronomy. 1 (1): 84–138. Bibcode:1955VA……1…84H. doi:10.1016/0083-6656(55)90016-7. at pp. 118–122.
- ^ Ansari, S. M. Razaullah (2002). History of oriental astronomy: proceedings of the joint discussion-17 at the 23rd General Assembly of the International Astronomical Union, organised by the Commission 41 (History of Astronomy), held in Kyoto, August 25–26, 1997. Springer Science+Business Media. p. 137. ISBN 1-4020-0657-8.
- ^ Goldstein, Bernard R. (1969). «Some Medieval Reports of Venus and Mercury Transits». Centaurus. 14 (1): 49–59. Bibcode:1969Cent…14…49G. doi:10.1111/j.1600-0498.1969.tb00135.x.
- ^ Ramasubramanian, K.; Srinivas, M. S.; Sriram, M. S. (1994). «Modification of the Earlier Indian Planetary Theory by the Kerala Astronomers (c. 1500 AD) and the Implied Heliocentric Picture of Planetary Motion» (PDF). Current Science. 66: 784–790. Archived from the original (PDF) on December 23, 2010. Retrieved April 23, 2010.
- ^ Gaab, Hans (2018). Simon Marius and His Research. Springer. p. 256. ISBN 978-3-319-92620-9.
Marius noted in the dedication from June 30, 1612, in the Prognosticon auf 1613 «that Mercury is illuminated by the Sun in the same way as the Venus and the Moon» and reports his observations of the brightness.
- ^ Sinnott, Roger W.; Meeus, Jean (1986). «John Bevis and a Rare Occultation». Sky and Telescope. 72: 220. Bibcode:1986S&T….72..220S.
- ^ Ferris, Timothy (2003). Seeing in the Dark: How Amateur Astronomers. Simon and Schuster. ISBN 978-0-684-86580-5.
- ^ a b Colombo, Giuseppe; Shapiro, Irwin I. (November 1965). «The Rotation of the Planet Mercury». SAO Special Report #188R. 188: 188. Bibcode:1965SAOSR.188…..C.
- ^ Holden, Edward S. (1890). «Announcement of the Discovery of the Rotation Period of Mercury [by Professor Schiaparelli]». Publications of the Astronomical Society of the Pacific. 2 (7): 79. Bibcode:1890PASP….2…79H. doi:10.1086/120099. S2CID 122095054.
- ^ Davies, Merton E.; Dwornik, Stephen E.; Gault, Donald E.; Strom, Robert G. (1978). «Surface Mapping». Atlas of Mercury. NASA Office of Space Sciences. Retrieved May 28, 2008.
- ^ Evans, John V.; Brockelman, Richard A.; Henry, John C.; Hyde, Gerald M.; Kraft, Leon G.; Reid, Wyatt A.; Smith, W. W. (1965). «Radio Echo Observations of Venus and Mercury at 23 cm Wavelength». Astronomical Journal. 70: 487–500. Bibcode:1965AJ…..70..486E. doi:10.1086/109772.
- ^ Moore, Patrick (2000). The Data Book of Astronomy. New York: CRC Press. p. 483. ISBN 978-0-7503-0620-1.
- ^ Butrica, Andrew J. (1996). «Chapter 5». To See the Unseen: A History of Planetary Radar Astronomy. NASA History Office, Washington D.C. ISBN 978-0-16-048578-7.
- ^ Pettengill, Gordon H.; Dyce, Rolf B. (1965). «A Radar Determination of the Rotation of the Planet Mercury». Nature. 206 (1240): 451–2. Bibcode:1965Natur.206Q1240P. doi:10.1038/2061240a0. S2CID 31525579.
- ^ «Mercury». Eric Weisstein’s World of Astronomy. Wolfram Research. Retrieved April 18, 2021.
- ^ Murray, Bruce C.; Burgess, Eric (1977). Flight to Mercury. Columbia University Press. ISBN 978-0-231-03996-3.
- ^ Colombo, Giuseppe (1965). «Rotational Period of the Planet Mercury». Nature. 208 (5010): 575. Bibcode:1965Natur.208..575C. doi:10.1038/208575a0. S2CID 4213296.
- ^ Davies, Merton E.; et al. (1976). «Mariner 10 Mission and Spacecraft». SP-423 Atlas of Mercury. NASA JPL. Retrieved April 7, 2008.
- ^ Golden, Leslie M. (1977). A Microwave Interferometric Study of the Subsurface of the Planet Mercury (Thesis). University of California, Berkeley. Bibcode:1977PhDT………9G.
- ^ Mitchell, David L.; De Pater, Imke (1994). «Microwave Imaging of Mercury’s Thermal Emission at Wavelengths from 0.3 to 20.5 cm (1994)». Icarus. 110 (1): 2–32. Bibcode:1994Icar..110….2M. doi:10.1006/icar.1994.1105.
- ^ Dantowitz, Ronald F.; Teare, Scott W.; Kozubal, Marek J. (2000). «Ground-based High-Resolution Imaging of Mercury». Astronomical Journal. 119 (4): 2455–2457. Bibcode:2000AJ….119.2455D. doi:10.1086/301328. S2CID 121483006.
- ^ Harmon, John K.; Slade, Martin A.; Butler, Bryan J.; Head III, James W.; Rice, Melissa S.; Campbell, Donald B. (2007). «Mercury: Radar images of the equatorial and midlatitude zones». Icarus. 187 (2): 374–405. Bibcode:2007Icar..187..374H. doi:10.1016/j.icarus.2006.09.026.
- ^ Webster, Guy (June 10, 2014). «Mercury Passes in Front of the Sun, as Seen From Mars». NASA. Retrieved June 10, 2014.
- ^ Zacny, Kris (July 2, 2015). Inner Solar System: Prospective Energy and Material Resources. Springer International Publishing. p. 154. ISBN 9783319195698.
- ^ Wagner, Sam; Wie, Bong (November 2015). «Hybrid Algorithm for Multiple Gravity-Assist and Impulsive Delta-V Maneuvers». Journal of Guidance, Control, and Dynamics. 38 (11): 2096–2107. Bibcode:2015JGCD…38.2096W. doi:10.2514/1.G000874.
- ^ «Mercury» (PDF). NASA Jet Propulsion Laboratory. May 5, 2008. Retrieved April 26, 2021.
- ^ Leipold, Manfred E.; Seboldt, W.; Lingner, Stephan; Borg, Erik; Herrmann, Axel Siegfried; Pabsch, Arno; Wagner, O.; Brückner, Johannes (1996). «Mercury sun-synchronous polar orbiter with a solar sail». Acta Astronautica. 39 (1): 143–151. Bibcode:1996AcAau..39..143L. doi:10.1016/S0094-5765(96)00131-2.
- ^ Dunne, James A. & Burgess, Eric (1978). «Chapter Four». The Voyage of Mariner 10 – Mission to Venus and Mercury. NASA History Office. Retrieved May 28, 2008.
- ^ Phillips, Tony (October 1976). «NASA 2006 Transit of Mercury». SP-423 Atlas of Mercury. NASA. Retrieved April 7, 2008.
- ^ «BepiColumbo – Background Science». European Space Agency. Retrieved June 18, 2017.
- ^ Malik, Tariq (August 16, 2004). «MESSENGER to test theory of shrinking Mercury». USA Today. Retrieved May 23, 2008.
- ^ Davies ME, et al. (1978). «Mariner 10 Mission and Spacecraft». Atlas of Mercury. NASA Office of Space Sciences. Retrieved May 30, 2008.
- ^ Ness, Norman F. (1978). «Mercury – Magnetic field and interior». Space Science Reviews. 21 (5): 527–553. Bibcode:1978SSRv…21..527N. doi:10.1007/BF00240907. S2CID 120025983.
- ^ Aharonson, Oded; Zuber, Maria T; Solomon, Sean C (2004). «Crustal remanence in an internally magnetized non-uniform shell: a possible source for Mercury’s magnetic field?». Earth and Planetary Science Letters. 218 (3–4): 261–268. Bibcode:2004E&PSL.218..261A. doi:10.1016/S0012-821X(03)00682-4.
- ^ Dunne, James A. & Burgess, Eric (1978). «Chapter Eight». The Voyage of Mariner 10 – Mission to Venus and Mercury. NASA History Office.
- ^ Grayzeck, Ed (April 2, 2008). «Mariner 10». NSSDC Master Catalog. NASA. Retrieved April 7, 2008.
- ^ «MESSENGER Engine Burn Puts Spacecraft on Track for Venus». SpaceRef.com. 2005. Retrieved March 2, 2006.
- ^ a b «Countdown to MESSENGER’s Closest Approach with Mercury». Johns Hopkins University Applied Physics Laboratory. January 14, 2008. Archived from the original on May 13, 2013. Retrieved May 30, 2008.
- ^ «MESSENGER Gains Critical Gravity Assist for Mercury Orbital Observations». MESSENGER Mission News. September 30, 2009. Archived from the original on May 10, 2013. Retrieved September 30, 2009.
- ^ «NASA extends spacecraft’s Mercury mission». United Press International. November 15, 2011. Retrieved November 16, 2011.
- ^ «MESSENGER: Fact Sheet» (PDF). Applied Physics Laboratory. February 2011. Retrieved August 21, 2017.
- ^ Wall, Mike (March 29, 2015). «NASA Mercury Probe Trying to Survive for Another Month». Space.com. Retrieved April 4, 2015.
- ^ Chang, Kenneth (April 27, 2015). «NASA’s Messenger Mission Is Set to Crash Into Mercury». The New York Times. Archived from the original on April 29, 2015. Retrieved April 27, 2015.
- ^ Corum, Jonathan (April 30, 2015). «Messenger’s Collision Course With Mercury». The New York Times. Retrieved April 30, 2015.
- ^ «Best Determination of MESSENGER’s Impact Location». MESSENGER Featured Images. Johns Hopkins – Applied Physics Lab. June 3, 2015. Retrieved April 29, 2015.
- ^ a b «ESA gives go-ahead to build BepiColombo». European Space Agency. February 26, 2007. Retrieved May 29, 2008.
- ^ «BepiColombo Fact Sheet». European Space Agency. December 1, 2016. Retrieved December 19, 2016.
- ^ «Objectives». European Space Agency. February 21, 2006. Retrieved May 29, 2008.
- ^ Warren, Haygen (October 24, 2021). «BepiColombo completes first Mercury flyby, science provides insight into planet’s unique environment». NASA Spaceflight. Retrieved October 8, 2022.
External links
This audio file was created from a revision of this article dated 16 January 2008, and does not reflect subsequent edits.
- Atlas of Mercury. NASA. 1978. SP-423.
- Mercury nomenclature and map with feature names from the USGS/IAU Gazetteer of Planetary Nomenclature
- Equirectangular map of Mercury by Applied Coherent Technology Corp
- 3D globe of Mercury by Google
- Mercury at Solarviews.com
- Mercury by Astronomy Cast
- MESSENGER mission web site
- BepiColombo mission web site
«First planet» redirects here. For other systems of numbering planets, see Planet § History.
![]() Mercury in true color (by MESSENGER in 2008) | ||||||||||||
Designations | ||||||||||||
---|---|---|---|---|---|---|---|---|---|---|---|---|
Pronunciation | (![]() | |||||||||||
Adjectives | Mercurian ,[1] Mercurial [2] | |||||||||||
Orbital characteristics[5] | ||||||||||||
Epoch J2000 | ||||||||||||
Aphelion |
| |||||||||||
Perihelion |
| |||||||||||
Semi-major axis |
| |||||||||||
Eccentricity | 0.205630[3] | |||||||||||
Orbital period (sidereal) |
| |||||||||||
Orbital period (synodic) | 115.88 d[3] | |||||||||||
Average orbital speed | 47.36 km/s[3] | |||||||||||
Mean anomaly | 174.796° | |||||||||||
Inclination |
| |||||||||||
Longitude of ascending node | 48.331° | |||||||||||
Argument of perihelion | 29.124° | |||||||||||
Satellites | None | |||||||||||
Physical characteristics | ||||||||||||
Mean diameter | 4880 km | |||||||||||
Mean radius |
| |||||||||||
Flattening | 0.0009[3] | |||||||||||
Surface area |
| |||||||||||
Volume |
| |||||||||||
Mass |
| |||||||||||
Mean density | 5.427 g/cm3[6] | |||||||||||
Surface gravity |
| |||||||||||
Moment of inertia factor | 0.346±0.014[9] | |||||||||||
Escape velocity | 4.25 km/s[6] | |||||||||||
Synodic rotation period | 176 d[10] | |||||||||||
Sidereal rotation period |
| |||||||||||
Equatorial rotation velocity | 10.892 km/h (3.026 m/s) | |||||||||||
Axial tilt | 2.04′ ± 0.08′ (to orbit)[9] (0.034°)[3] | |||||||||||
North pole right ascension |
| |||||||||||
North pole declination | 61.45°[3] | |||||||||||
Albedo |
| |||||||||||
Temperature | 437 K (164 °C) (blackbody temperature)[13] | |||||||||||
| ||||||||||||
Apparent magnitude | −2.48 to +7.25[15] | |||||||||||
Angular diameter | 4.5–13″[3] | |||||||||||
Atmosphere[3][16][17] | ||||||||||||
Surface pressure | trace (≲ 0.5 nPa) | |||||||||||
Composition by volume |
| |||||||||||
Mercury is the smallest planet in the Solar System and the closest to the Sun. Its orbit around the Sun takes 87.97 Earth days, the shortest of all the Sun’s planets. It is named after the Roman god Mercurius (Mercury), god of commerce, messenger of the gods, and mediator between gods and mortals, corresponding to the Greek god Hermes (Ἑρμῆς). Like Venus, Mercury orbits the Sun within Earth’s orbit as an inferior planet; its apparent distance from the Sun as viewed from Earth never exceeds 28°. This proximity to the Sun means the planet can only be seen near the western horizon after sunset or the eastern horizon before sunrise, usually in twilight. At this time, it may appear as a bright star-like object, but is more difficult to observe than Venus. From Earth, the planet telescopically displays the complete range of phases, similar to Venus and the Moon, which recurs over its synodic period of approximately 116 days. Due to its synodic proximity to Earth, Mercury is most often the closest planet to Earth, with Venus periodically taking this role.[18][19]
Mercury rotates in a way that is unique in the Solar System. It is tidally locked with the Sun in a 3:2 spin–orbit resonance,[20] meaning that relative to the fixed stars, it rotates on its axis exactly three times for every two revolutions it makes around the Sun.[a][21] As seen from the Sun, in a frame of reference that rotates with the orbital motion, it appears to rotate only once every two Mercurian years. An observer on Mercury would therefore see only one day every two Mercurian years.
Mercury’s axis has the smallest tilt of any of the Solar System’s planets (about 1⁄30 degree). Its orbital eccentricity is the largest of all known planets in the Solar System;[b] at perihelion, Mercury’s distance from the Sun is only about two-thirds (or 66%) of its distance at aphelion. Mercury’s surface appears heavily cratered and is similar in appearance to the Moon’s, indicating that it has been geologically inactive for billions of years. Having almost no atmosphere to retain heat, it has surface temperatures that vary diurnally more than on any other planet in the Solar System, ranging from 100 K (−173 °C; −280 °F) at night to 700 K (427 °C; 800 °F) during the day across the equatorial regions.[22] The polar regions are constantly below 180 K (−93 °C; −136 °F). The planet has no natural satellites.
Two spacecraft have visited Mercury: Mariner 10 flew by in 1974 and 1975; and MESSENGER, launched in 2004, orbited Mercury over 4,000 times in four years before exhausting its fuel and crashing into the planet’s surface on April 30, 2015.[23][24][25] The BepiColombo spacecraft is planned to arrive at Mercury in 2025.
Nomenclature
The ancients knew Mercury by different names depending on whether it was an evening star or a morning star. By about 350 BC, the ancient Greeks had realized the two stars were one.[26] They knew the planet as Στίλβων Stilbōn, meaning «twinkling», and Ἑρμής Hermēs, for its fleeting motion,[27] a name that is retained in modern Greek (Ερμής Ermis).[28] The Romans named the planet after the swift-footed Roman messenger god, Mercury (Latin Mercurius), which they equated with the Greek Hermes, because it moves across the sky faster than any other planet.[26][29] The astronomical symbol for Mercury is a stylized version of Hermes’ caduceus; a Christian cross was added in the 16th century: .[30][31]
Physical characteristics
Mercury is one of four terrestrial planets in the Solar System, and is a rocky body like Earth. It is the smallest planet in the Solar System, with an equatorial radius of 2,439.7 kilometres (1,516.0 mi).[3] Mercury is also smaller—albeit more massive—than the largest natural satellites in the Solar System, Ganymede and Titan. Mercury consists of approximately 70% metallic and 30% silicate material.[32]
Internal structure
Mercury’s internal structure and magnetic field
Mercury appears to have a solid silicate crust and mantle overlying a solid, iron sulfide outer core layer, a deeper liquid core layer, and a solid inner core.[33][34] The planet’s density is the second highest in the Solar System at 5.427 g/cm3, only slightly less than Earth’s density of 5.515 g/cm3.[3] If the effect of gravitational compression were to be factored out from both planets, the materials of which Mercury is made would be denser than those of Earth, with an uncompressed density of 5.3 g/cm3 versus Earth’s 4.4 g/cm3.[35] Mercury’s density can be used to infer details of its inner structure. Although Earth’s high density results appreciably from gravitational compression, particularly at the core, Mercury is much smaller and its inner regions are not as compressed. Therefore, for it to have such a high density, its core must be large and rich in iron.[36]
The radius of Mercury’s core is estimated to be 2,020 ± 30 km (1,255 ± 19 mi), based on interior models constrained to be consistent with the value of the moment of inertia factor given in the infobox.[9][37] Hence, Mercury’s core occupies about 57% of its volume; for Earth this proportion is 17%. Research published in 2007 suggests that Mercury has a molten core.[38][39] Surrounding the core is a 500–700 km (310–430 mi) mantle consisting of silicates.[40][41] Based on data from the Mariner 10 and MESSENGER missions, in addition to Earth-based observation, Mercury’s crust is estimated to be 35 km (22 mi) thick.[42][43] However, this model may be an overestimate and the crust could be 26 ± 11 km (16.2 ± 6.8 mi) thick based on an Airy isostacy model.[44] One distinctive feature of Mercury’s surface is the presence of numerous narrow ridges, extending up to several hundred kilometers in length. It is thought that these were formed as Mercury’s core and mantle cooled and contracted at a time when the crust had already solidified.[45][46][47]
Mercury’s core has a higher iron content than that of any other major planet in the Solar System, and several theories have been proposed to explain this. The most widely accepted theory is that Mercury originally had a metal–silicate ratio similar to common chondrite meteorites, thought to be typical of the Solar System’s rocky matter, and a mass approximately 2.25 times its current mass.[48] Early in the Solar System’s history, Mercury may have been struck by a planetesimal of approximately 1/6 Mercury’s mass and several thousand kilometers across.[48] The impact would have stripped away much of the original crust and mantle, leaving the core behind as a relatively major component.[48] A similar process, known as the giant impact hypothesis, has been proposed to explain the formation of the Moon.[48]
Alternatively, Mercury may have formed from the solar nebula before the Sun’s energy output had stabilized. It would initially have had twice its present mass, but as the protosun contracted, temperatures near Mercury could have been between 2,500 and 3,500 K and possibly even as high as 10,000 K.[49] Much of Mercury’s surface rock could have been vaporized at such temperatures, forming an atmosphere of «rock vapor» that could have been carried away by the solar wind.[49] A third hypothesis proposes that the solar nebula caused drag on the particles from which Mercury was accreting, which meant that lighter particles were lost from the accreting material and not gathered by Mercury.[50]
Each hypothesis predicts a different surface composition, and two space missions have been tasked with making observations of this composition. The first MESSENGER, which ended in 2015, found higher-than-expected potassium and sulfur levels on the surface, suggesting that the giant impact hypothesis and vaporization of the crust and mantle did not occur because said potassium and sulfur would have been driven off by the extreme heat of these events.[51] BepiColombo, which will arrive at Mercury in 2025, will make observations to test these hypotheses.[52] The findings so far would seem to favor the third hypothesis; however, further analysis of the data is needed.[53]
Surface geology
Mercury’s surface is similar in appearance to that of the Moon, showing extensive mare-like plains and heavy cratering, indicating that it has been geologically inactive for billions of years. It is more heterogeneous than the surface of Mars or the Moon, both of which contain significant stretches of similar geology, such as maria and plateaus.[54] Albedo features are areas of markedly different reflectivity, which include impact craters, the resulting ejecta, and ray systems. Larger albedo features correspond to higher reflectivity plains.[55] Mercury has dorsa (also called «wrinkle-ridges»), Moon-like highlands, montes (mountains), planitiae (plains), rupes (escarpments), and valles (valleys).[56][57]
MASCS spectrum scan of Mercury’s surface by MESSENGER
The planet’s mantle is chemically heterogeneous, suggesting the planet went through a magma ocean phase early in its history. Crystallization of minerals and convective overturn resulted in layered, chemically heterogeneous crust with large-scale variations in chemical composition observed on the surface. The crust is low in iron but high in sulfur, resulting from the stronger early chemically reducing conditions than is found in the other terrestrial planets. The surface is dominated by iron-poor pyroxene and olivine, as represented by enstatite and forsterite, respectively, along with sodium-rich plagioclase and minerals of mixed magnesium, calcium, and iron-sulfide. The less reflective regions of the crust are high in carbon, most likely in the form of graphite.[58]
Names for features on Mercury come from a variety of sources. Names coming from people are limited to the deceased. Craters are named for artists, musicians, painters, and authors who have made outstanding or fundamental contributions to their field. Ridges, or dorsa, are named for scientists who have contributed to the study of Mercury. Depressions or fossae are named for works of architecture. Montes are named for the word «hot» in a variety of languages. Plains or planitiae are named for Mercury in various languages. Escarpments or rupēs are named for ships of scientific expeditions. Valleys or valles are named for abandoned cities, towns, or settlements of antiquity.[59]
Impact basins and craters
Mercury was heavily bombarded by comets and asteroids during and shortly following its formation 4.6 billion years ago, as well as during a possibly separate subsequent episode called the Late Heavy Bombardment that ended 3.8 billion years ago.[60] Mercury received impacts over its entire surface during this period of intense crater formation,[57] facilitated by the lack of any atmosphere to slow impactors down.[61] During this time Mercury was volcanically active; basins were filled by magma, producing smooth plains similar to the maria found on the Moon.[62][63] One of the most unusual craters is Apollodorus, or «the Spider», which hosts a serious of radiating troughs extending outwards from its impact site.[64]
Craters on Mercury range in diameter from small bowl-shaped cavities to multi-ringed impact basins hundreds of kilometers across. They appear in all states of degradation, from relatively fresh rayed craters to highly degraded crater remnants. Mercurian craters differ subtly from lunar craters in that the area blanketed by their ejecta is much smaller, a consequence of Mercury’s stronger surface gravity.[65] According to International Astronomical Union rules, each new crater must be named after an artist who was famous for more than fifty years, and dead for more than three years, before the date the crater is named.[66]
Overhead view of Caloris Basin
Perspective view of Caloris Basin – high (red); low (blue)
The largest known crater is Caloris Planitia, or Caloris Basin, with a diameter of 1,550 km.[67] The impact that created the Caloris Basin was so powerful that it caused lava eruptions and left a concentric mountainous ring ~2 km tall surrounding the impact crater. The floor of the Caloris Basin is filled by a geologically distinct flat plain, broken up by ridges and fractures in a roughly polygonal pattern. It is not clear whether they are volcanic lava flows induced by the impact or a large sheet of impact melt.[65]
At the antipode of the Caloris Basin is a large region of unusual, hilly terrain known as the «Weird Terrain». One hypothesis for its origin is that shock waves generated during the Caloris impact traveled around Mercury, converging at the basin’s antipode (180 degrees away). The resulting high stresses fractured the surface.[68] Alternatively, it has been suggested that this terrain formed as a result of the convergence of ejecta at this basin’s antipode.[69]
Tolstoj basin is along the bottom of this image of Mercury’s limb
Overall, 46 impact basins have been identified.[70] A notable basin is the 400 km wide, multi-ring Tolstoj Basin that has an ejecta blanket extending up to 500 km from its rim and a floor that has been filled by smooth plains materials. Beethoven Basin has a similar-sized ejecta blanket and a 625 km diameter rim.[65] Like the Moon, the surface of Mercury has likely incurred the effects of space weathering processes, including solar wind and micrometeorite impacts.[71]
Plains
There are two geologically distinct plains regions on Mercury.[65][72] Gently rolling, hilly plains in the regions between craters are Mercury’s oldest visible surfaces,[65] predating the heavily cratered terrain. These inter-crater plains appear to have obliterated many earlier craters, and show a general paucity of smaller craters below about 30 km in diameter.[72]
Smooth plains are widespread flat areas that fill depressions of various sizes and bear a strong resemblance to the lunar maria. Unlike lunar maria, the smooth plains of Mercury have the same albedo as the older inter-crater plains. Despite a lack of unequivocally volcanic characteristics, the localisation and rounded, lobate shape of these plains strongly support volcanic origins.[65] All the smooth plains of Mercury formed significantly later than the Caloris basin, as evidenced by appreciably smaller crater densities than on the Caloris ejecta blanket.[65]
Compressional features
One unusual feature of Mercury’s surface is the numerous compression folds, or rupes, that crisscross the plains. These also exist on the moon, but are much more prominent on Mercury.[73] As Mercury’s interior cooled, it contracted and its surface began to deform, creating wrinkle ridges and lobate scarps associated with thrust faults. The scarps can reach lengths of 1000 km and heights of 3 km.[74] These compressional features can be seen on top of other features, such as craters and smooth plains, indicating they are more recent.[75] Mapping of the features has suggested a total shrinkage of Mercury’s radius in the range of ~1 to 7 km.[76] Most activity along the major thrust systems probably ended about 3.6–3.7 billion years ago.[77] Small-scale thrust fault scarps have been found, tens of meters in height and with lengths in the range of a few km, that appear to be less than 50 million years old, indicating that compression of the interior and consequent surface geological activity continue to the present.[74][76]
Volcanism
Picasso crater — the large arc-shaped pit located on the eastern side of its floor are postulated to have formed when subsurface magma subsided or drained, causing the surface to collapse into the resulting void.
There is evidence for pyroclastic flows on Mercury from low-profile shield volcanoes.[78][79][80] 51 pyroclastic deposits have been identified,[81] where 90% of them are found within impact craters.[81] A study of the degradation state of the impact craters that host pyroclastic deposits suggests that pyroclastic activity occurred on Mercury over a prolonged interval.[81]
A «rimless depression» inside the southwest rim of the Caloris Basin consists of at least nine overlapping volcanic vents, each individually up to 8 km in diameter. It is thus a «compound volcano».[82] The vent floors are at least 1 km below their brinks and they bear a closer resemblance to volcanic craters sculpted by explosive eruptions or modified by collapse into void spaces created by magma withdrawal back down into a conduit.[82] Scientists could not quantify the age of the volcanic complex system but reported that it could be on the order of a billion years.[82]
Surface conditions and exosphere
Composite of the north pole of Mercury, where NASA confirmed the discovery of a large volume of water ice, in permanently dark craters that are found there.[83]
The surface temperature of Mercury ranges from 100 to 700 K (−173 to 427 °C; −280 to 800 °F)[22] at the most extreme places: 0°N, 0°W, or 180°W. It never rises above 180 K at the poles,[14] due to the absence of an atmosphere and a steep temperature gradient between the equator and the poles. The subsolar point reaches about 700 K during perihelion (0°W or 180°W), but only 550 K at aphelion (90° or 270°W).[84]
On the dark side of the planet, temperatures average 110 K.[14][85]
The intensity of sunlight on Mercury’s surface ranges between 4.59 and 10.61 times the solar constant (1,370 W·m−2).[86]
Although the daylight temperature at the surface of Mercury is generally extremely high, observations strongly suggest that ice (frozen water) exists on Mercury. The floors of deep craters at the poles are never exposed to direct sunlight, and temperatures there remain below 102 K, far lower than the global average.[87] This creates a cold trap where ice can accumulate. Water ice strongly reflects radar, and observations by the 70-meter Goldstone Solar System Radar and the VLA in the early 1990s revealed that there are patches of high radar reflection near the poles.[88] Although ice was not the only possible cause of these reflective regions, astronomers think it was the most likely.[89]
The icy regions are estimated to contain about 1014–1015 kg of ice,[90] and may be covered by a layer of regolith that inhibits sublimation.[91] By comparison, the Antarctic ice sheet on Earth has a mass of about 4×1018 kg, and Mars’s south polar cap contains about 1016 kg of water.[90] The origin of the ice on Mercury is not yet known, but the two most likely sources are from outgassing of water from the planet’s interior or deposition by impacts of comets.[90]
Mercury is too small and hot for its gravity to retain any significant atmosphere over long periods of time; it does have a tenuous surface-bounded exosphere[92] containing hydrogen, helium, oxygen, sodium, calcium, potassium and others[16][17] at a surface pressure of less than approximately 0.5 nPa (0.005 picobars).[3] This exosphere is not stable—atoms are continuously lost and replenished from a variety of sources. Hydrogen atoms and helium atoms probably come from the solar wind, diffusing into Mercury’s magnetosphere before later escaping back into space. Radioactive decay of elements within Mercury’s crust is another source of helium, as well as sodium and potassium. MESSENGER found high proportions of calcium, helium, hydroxide, magnesium, oxygen, potassium, silicon and sodium. Water vapor is present, released by a combination of processes such as: comets striking its surface, sputtering creating water out of hydrogen from the solar wind and oxygen from rock, and sublimation from reservoirs of water ice in the permanently shadowed polar craters. The detection of high amounts of water-related ions like O+, OH−, and H3O+ was a surprise.[93][94] Because of the quantities of these ions that were detected in Mercury’s space environment, scientists surmise that these molecules were blasted from the surface or exosphere by the solar wind.[95][96]
Sodium, potassium and calcium were discovered in the atmosphere during the 1980–1990s, and are thought to result primarily from the vaporization of surface rock struck by micrometeorite impacts[97] including presently from Comet Encke.[98] In 2008, magnesium was discovered by MESSENGER.[99] Studies indicate that, at times, sodium emissions are localized at points that correspond to the planet’s magnetic poles. This would indicate an interaction between the magnetosphere and the planet’s surface.[100]
On November 29, 2012, NASA confirmed that images from MESSENGER had detected that craters at the north pole contained water ice. MESSENGER‘s principal investigator Sean Solomon is quoted in The New York Times estimating the volume of the ice to be large enough to «encase Washington, D.C., in a frozen block two and a half miles deep».[83]
According to NASA, Mercury is not a suitable planet for Earth-like life. It has a surface boundary exosphere instead of a layered atmosphere, extreme temperatures, and high solar radiation. It is unlikely that any living beings can withstand those conditions.[101] Some parts of the subsurface of Mercury may have been habitable, and perhaps life forms, albeit likely primitive microorganisms, may have existed on the planet.[102][103][104]
Magnetic field and magnetosphere
Graph showing relative strength of Mercury’s magnetic field
Despite its small size and slow 59-day-long rotation, Mercury has a significant, and apparently global, magnetic field. According to measurements taken by Mariner 10, it is about 1.1% the strength of Earth’s. The magnetic-field strength at Mercury’s equator is about 300 nT.[105][106] Like that of Earth, Mercury’s magnetic field is dipolar.[100] Unlike Earth’s, Mercury’s poles are nearly aligned with the planet’s spin axis.[107] Measurements from both the Mariner 10 and MESSENGER space probes have indicated that the strength and shape of the magnetic field are stable.[107]
It is likely that this magnetic field is generated by a dynamo effect, in a manner similar to the magnetic field of Earth.[108][109] This dynamo effect would result from the circulation of the planet’s iron-rich liquid core. Particularly strong tidal heating effects caused by the planet’s high orbital eccentricity would serve to keep part of the core in the liquid state necessary for this dynamo effect.[40][110]
Mercury’s magnetic field is strong enough to deflect the solar wind around the planet, creating a magnetosphere. The planet’s magnetosphere, though small enough to fit within Earth,[100] is strong enough to trap solar wind plasma. This contributes to the space weathering of the planet’s surface.[107] Observations taken by the Mariner 10 spacecraft detected this low energy plasma in the magnetosphere of the planet’s nightside. Bursts of energetic particles in the planet’s magnetotail indicate a dynamic quality to the planet’s magnetosphere.[100]
During its second flyby of the planet on October 6, 2008, MESSENGER discovered that Mercury’s magnetic field can be extremely «leaky». The spacecraft encountered magnetic «tornadoes» – twisted bundles of magnetic fields connecting the planetary magnetic field to interplanetary space – that were up to 800 km wide or a third of the radius of the planet. These twisted magnetic flux tubes, technically known as flux transfer events, form open windows in the planet’s magnetic shield through which the solar wind may enter and directly impact Mercury’s surface via magnetic reconnection[111] This also occurs in Earth’s magnetic field. The MESSENGER observations showed the reconnection rate is ten times higher at Mercury, but its proximity to the Sun only accounts for about a third of the reconnection rate observed by MESSENGER.[111]
Orbit, rotation, and longitude
Orbit of Mercury (2006)
Animation of Mercury’s and Earth’s revolution around the Sun
Mercury has the most eccentric orbit of all the planets in the Solar System; its eccentricity is 0.21 with its distance from the Sun ranging from 46,000,000 to 70,000,000 km (29,000,000 to 43,000,000 mi). It takes 87.969 Earth days to complete an orbit. The diagram illustrates the effects of the eccentricity, showing Mercury’s orbit overlaid with a circular orbit having the same semi-major axis. Mercury’s higher velocity when it is near perihelion is clear from the greater distance it covers in each 5-day interval. In the diagram, the varying distance of Mercury to the Sun is represented by the size of the planet, which is inversely proportional to Mercury’s distance from the Sun. This varying distance to the Sun leads to Mercury’s surface being flexed by tidal bulges raised by the Sun that are about 17 times stronger than the Moon’s on Earth.[112] Combined with a 3:2 spin–orbit resonance of the planet’s rotation around its axis, it also results in complex variations of the surface temperature.[32]
The resonance makes a single solar day (the length between two meridian transits of the Sun) on Mercury last exactly two Mercury years, or about 176 Earth days.[113]
Mercury’s orbit is inclined by 7 degrees to the plane of Earth’s orbit (the ecliptic), the largest of all eight known solar planets.[114] As a result, transits of Mercury across the face of the Sun can only occur when the planet is crossing the plane of the ecliptic at the time it lies between Earth and the Sun, which is in May or November. This occurs about every seven years on average.[115]
Mercury’s axial tilt is almost zero,[116] with the best measured value as low as 0.027 degrees.[117] This is significantly smaller than that of Jupiter, which has the second smallest axial tilt of all planets at 3.1 degrees. This means that to an observer at Mercury’s poles, the center of the Sun never rises more than 2.1 arcminutes above the horizon.[117]
At certain points on Mercury’s surface, an observer would be able to see the Sun peek up a little more than two-thirds of the way over the horizon, then reverse and set before rising again, all within the same Mercurian day.[c] This is because approximately four Earth days before perihelion, Mercury’s angular orbital velocity equals its angular rotational velocity so that the Sun’s apparent motion ceases; closer to perihelion, Mercury’s angular orbital velocity then exceeds the angular rotational velocity. Thus, to a hypothetical observer on Mercury, the Sun appears to move in a retrograde direction. Four Earth days after perihelion, the Sun’s normal apparent motion resumes.[32] A similar effect would have occurred if Mercury had been in synchronous rotation: the alternating gain and loss of rotation over revolution would have caused a libration of 23.65° in longitude.[118]
For the same reason, there are two points on Mercury’s equator, 180 degrees apart in longitude, at either of which, around perihelion in alternate Mercurian years (once a Mercurian day), the Sun passes overhead, then reverses its apparent motion and passes overhead again, then reverses a second time and passes overhead a third time, taking a total of about 16 Earth-days for this entire process. In the other alternate Mercurian years, the same thing happens at the other of these two points. The amplitude of the retrograde motion is small, so the overall effect is that, for two or three weeks, the Sun is almost stationary overhead, and is at its most brilliant because Mercury is at perihelion, its closest to the Sun. This prolonged exposure to the Sun at its brightest makes these two points the hottest places on Mercury. Maximum temperature occurs when the Sun is at an angle of about 25 degrees past noon due to diurnal temperature lag, at 0.4 Mercury days and 0.8 Mercury years past sunrise.[119] Conversely, there are two other points on the equator, 90 degrees of longitude apart from the first ones, where the Sun passes overhead only when the planet is at aphelion in alternate years, when the apparent motion of the Sun in Mercury’s sky is relatively rapid. These points, which are the ones on the equator where the apparent retrograde motion of the Sun happens when it is crossing the horizon as described in the preceding paragraph, receive much less solar heat than the first ones described above.[120]
Mercury attains inferior conjunction (nearest approach to Earth) every 116 Earth days on average,[3] but this interval can range from 105 days to 129 days due to the planet’s eccentric orbit. Mercury can come as near as 82,200,000 km (0.549 astronomical units; 51.1 million miles) to Earth, and that is slowly declining: The next approach to within 82,100,000 km (51 million mi) is in 2679, and to within 82,000,000 km (51 million mi) in 4487, but it will not be closer to Earth than 80,000,000 km (50 million mi) until 28,622.[121] Its period of retrograde motion as seen from Earth can vary from 8 to 15 days on either side of inferior conjunction. This large range arises from the planet’s high orbital eccentricity.[32] Essentially because Mercury is closest to the Sun, when taking an average over time, Mercury is most often the closest planet to the Earth,[18][19] and—in that measure—it is the closest planet to each of the other planets in the Solar System.[122][123][124][d]
Longitude convention
The longitude convention for Mercury puts the zero of longitude at one of the two hottest points on the surface, as described above. However, when this area was first visited, by Mariner 10, this zero meridian was in darkness, so it was impossible to select a feature on the surface to define the exact position of the meridian. Therefore, a small crater further west was chosen, called Hun Kal, which provides the exact reference point for measuring longitude.[125][126] The center of Hun Kal defines the 20° west meridian. A 1970 International Astronomical Union resolution suggests that longitudes be measured positively in the westerly direction on Mercury.[127] The two hottest places on the equator are therefore at longitudes 0° W and 180° W, and the coolest points on the equator are at longitudes 90° W and 270° W. However, the MESSENGER project uses an east-positive convention.[128]
Spin-orbit resonance
After one orbit, Mercury has rotated 1.5 times, so after two complete orbits the same hemisphere is again illuminated.
For many years it was thought that Mercury was synchronously tidally locked with the Sun, rotating once for each orbit and always keeping the same face directed towards the Sun, in the same way that the same side of the Moon always faces Earth. Radar observations in 1965 proved that the planet has a 3:2 spin-orbit resonance, rotating three times for every two revolutions around the Sun. The eccentricity of Mercury’s orbit makes this resonance stable—at perihelion, when the solar tide is strongest, the Sun is nearly still in Mercury’s sky.[129]
The rare 3:2 resonant tidal locking is stabilized by the variance of the tidal force along Mercury’s eccentric orbit, acting on a permanent dipole component of Mercury’s mass distribution.[130] In a circular orbit there is no such variance, so the only resonance stabilized in such an orbit is at 1:1 (e.g., Earth–Moon), when the tidal force, stretching a body along the «center-body» line, exerts a torque that aligns the body’s axis of least inertia (the «longest» axis, and the axis of the aforementioned dipole) to point always at the center. However, with noticeable eccentricity, like that of Mercury’s orbit, the tidal force has a maximum at perihelion and therefore stabilizes resonances, like 3:2, ensuring that the planet points its axis of least inertia roughly at the Sun when passing through perihelion.[130]
The original reason astronomers thought it was synchronously locked was that, whenever Mercury was best placed for observation, it was always nearly at the same point in its 3:2 resonance, hence showing the same face. This is because, coincidentally, Mercury’s rotation period is almost exactly half of its synodic period with respect to Earth. Due to Mercury’s 3:2 spin-orbit resonance, a solar day lasts about 176 Earth days.[32] A sidereal day (the period of rotation) lasts about 58.7 Earth days.[32]
Simulations indicate that the orbital eccentricity of Mercury varies chaotically from nearly zero (circular) to more than 0.45 over millions of years due to perturbations from the other planets.[32][131]
This was thought to explain Mercury’s 3:2 spin-orbit resonance (rather than the more usual 1:1), because this state is more likely to arise during a period of high eccentricity.[132]
However, accurate modeling based on a realistic model of tidal response has demonstrated that Mercury was captured into the 3:2 spin-orbit state at a very early stage of its history, within 20 (more likely, 10) million years after its formation.[133]
Numerical simulations show that a future secular orbital resonant perihelion interaction with Jupiter may cause the eccentricity of Mercury’s orbit to increase to the point where there is a 1% chance that the orbit will be destabilized in the next five billion years. If this happens, Mercury may fall into the Sun, collide with Venus, be ejected from the Solar System, or even disrupt the rest of the inner Solar System.[134][135]
Advance of perihelion
In 1859, the French mathematician and astronomer Urbain Le Verrier reported that the slow precession of Mercury’s orbit around the Sun could not be completely explained by Newtonian mechanics and perturbations by the known planets. He suggested, among possible explanations, that another planet (or perhaps instead a series of smaller «corpuscules») might exist in an orbit even closer to the Sun than that of Mercury, to account for this perturbation.[136] (Other explanations considered included a slight oblateness of the Sun.) The success of the search for Neptune based on its perturbations of the orbit of Uranus led astronomers to place faith in this possible explanation, and the hypothetical planet was named Vulcan, but no such planet was ever found.[137]
The perihelion precession of Mercury is 5,600 arcseconds (1.5556°) per century relative to Earth, or 574.10±0.65 arcseconds per century[138] relative to the inertial ICRF. Newtonian mechanics, taking into account all the effects from the other planets, predicts a precession of 5,557 arcseconds (1.5436°) per century.[138] In the early 20th century, Albert Einstein’s general theory of relativity provided the explanation for the observed precession, by formalizing gravitation as being mediated by the curvature of spacetime. The effect is small: just 42.98 arcseconds per century for Mercury; it therefore requires a little over twelve million orbits for a full excess turn. Similar, but much smaller, effects exist for other Solar System bodies: 8.62 arcseconds per century for Venus, 3.84 for Earth, 1.35 for Mars, and 10.05 for 1566 Icarus.[139][140]
Observation
Image mosaic by Mariner 10, 1974
Mercury’s apparent magnitude is calculated to vary between −2.48 (brighter than Sirius) around superior conjunction and +7.25 (below the limit of naked-eye visibility) around inferior conjunction.[15] The mean apparent magnitude is 0.23 while the standard deviation of 1.78 is the largest of any planet. The mean apparent magnitude at superior conjunction is −1.89 while that at inferior conjunction is +5.93.[15] Observation of Mercury is complicated by its proximity to the Sun, as it is lost in the Sun’s glare for much of the time. Mercury can be observed for only a brief period during either morning or evening twilight.[141]
But in some cases Mercury can better be observed in daylight with a telescope when the position is known because it is higher in the sky and less atmospheric effects affect the view of the planet. When proper safety precautions are taken to prevent inadvertently pointing the telescope at the Sun (and thus blinding the user), Mercury can be viewed as close as 4° to the Sun when near superior conjunction when it is almost at its brightest.
Mercury can, like several other planets and the brightest stars, be seen during a total solar eclipse.[142]
Like the Moon and Venus, Mercury exhibits phases as seen from Earth. It is «new» at inferior conjunction and «full» at superior conjunction. The planet is rendered invisible from Earth on both of these occasions because of its being obscured by the Sun,[141] except its new phase during a transit.
Mercury is technically brightest as seen from Earth when it is at a full phase. Although Mercury is farthest from Earth when it is full, the greater illuminated area that is visible and the opposition brightness surge more than compensates for the distance.[143] The opposite is true for Venus, which appears brightest when it is a crescent, because it is much closer to Earth than when gibbous.[143][144]
False-color map showing the maximum temperatures of the north polar region
Nonetheless, the brightest (full phase) appearance of Mercury is an essentially impossible time for practical observation, because of the extreme proximity of the Sun. Mercury is best observed at the first and last quarter, although they are phases of lesser brightness. The first and last quarter phases occur at greatest elongation east and west of the Sun, respectively. At both of these times Mercury’s separation from the Sun ranges anywhere from 17.9° at perihelion to 27.8° at aphelion.[145][146] At greatest western elongation, Mercury rises at its earliest before sunrise, and at greatest eastern elongation, it sets at its latest after sunset.[147]
False-color image of Carnegie Rupes, a tectonic landform—high terrain (red); low (blue).
Mercury is more often and easily visible from the Southern Hemisphere than from the Northern. This is because Mercury’s maximum western elongation occurs only during early autumn in the Southern Hemisphere, whereas its greatest eastern elongation happens only during late winter in the Southern Hemisphere.[147] In both of these cases, the angle at which the planet’s orbit intersects the horizon is maximized, allowing it to rise several hours before sunrise in the former instance and not set until several hours after sundown in the latter from southern mid-latitudes, such as Argentina and South Africa.[147]
An alternate method for viewing Mercury involves observing the planet during daylight hours when conditions are clear, ideally when it is at its greatest elongation. This allows the planet to be found easily, even when using telescopes with 8 cm (3.1 in) apertures. However, great care must be taken to obstruct the Sun from sight because of the extreme risk for eye damage.[148] This method bypasses the limitation of twilight observing when the ecliptic is located at a low elevation (e.g. on autumn evenings).
Ground-based telescope observations of Mercury reveal only an illuminated partial disk with limited detail. The first of two spacecraft to visit the planet was Mariner 10, which mapped about 45% of its surface from 1974 to 1975. The second is the MESSENGER spacecraft, which after three Mercury flybys between 2008 and 2009, attained orbit around Mercury on March 17, 2011,[149] to study and map the rest of the planet.[150]
The Hubble Space Telescope cannot observe Mercury at all, due to safety procedures that prevent its pointing too close to the Sun.[151]
Because the shift of 0.15 revolutions in a year makes up a seven-year cycle (0.15 × 7 ≈ 1.0), in the seventh year Mercury follows almost exactly (earlier by 7 days) the sequence of phenomena it showed seven years before.[145]
Observation history
Ancient astronomers
Mercury, from Liber astronomiae, 1550
The earliest known recorded observations of Mercury are from the MUL.APIN tablets. These observations were most likely made by an Assyrian astronomer around the 14th century BC.[152] The cuneiform name used to designate Mercury on the MUL.APIN tablets is transcribed as UDU.IDIM.GUU4.UD («the jumping planet»).[e][153] Babylonian records of Mercury date back to the 1st millennium BC. The Babylonians called the planet Nabu after the messenger to the gods in their mythology.[154]
The Greco-Egyptian[155] astronomer Ptolemy wrote about the possibility of planetary transits across the face of the Sun in his work Planetary Hypotheses. He suggested that no transits had been observed either because planets such as Mercury were too small to see, or because the transits were too infrequent.[156]
In ancient China, Mercury was known as «the Hour Star» (Chen-xing 辰星). It was associated with the direction north and the phase of water in the Five Phases system of metaphysics.[157] Modern Chinese, Korean, Japanese and Vietnamese cultures refer to the planet literally as the «water star» (水星), based on the Five elements.[158][159][160] Hindu mythology used the name Budha for Mercury, and this god was thought to preside over Wednesday.[161] The god Odin (or Woden) of Germanic paganism was associated with the planet Mercury and Wednesday.[162] The Maya may have represented Mercury as an owl (or possibly four owls; two for the morning aspect and two for the evening) that served as a messenger to the underworld.[163]
In medieval Islamic astronomy, the Andalusian astronomer Abū Ishāq Ibrāhīm al-Zarqālī in the 11th century described the deferent of Mercury’s geocentric orbit as being oval, like an egg or a pignon, although this insight did not influence his astronomical theory or his astronomical calculations.[164][165] In the 12th century, Ibn Bajjah observed «two planets as black spots on the face of the Sun», which was later suggested as the transit of Mercury and/or Venus by the Maragha astronomer Qotb al-Din Shirazi in the 13th century.[166] (Note that most such medieval reports of transits were later taken as observations of sunspots.[167])
In India, the Kerala school astronomer Nilakantha Somayaji in the 15th century developed a partially heliocentric planetary model in which Mercury orbits the Sun, which in turn orbits Earth, similar to the Tychonic system later proposed by Tycho Brahe in the late 16th century.[168]
Ground-based telescopic research
Transit of Mercury. Mercury is visible as a black dot below and to the left of center. The dark area above the center of the solar disk is a sunspot.
Elongation is the angle between the Sun and the planet, with Earth as the reference point. Mercury appears close to the Sun.
The first telescopic observations of Mercury were made by Thomas Harriot and Galileo from 1610. In 1612, Simon Marius observed the brightness of Mercury varied with the planet’s orbital position and concluded it had phases «in the same way as Venus and the Moon».[169] In 1631, Pierre Gassendi made the first telescopic observations of the transit of a planet across the Sun when he saw a transit of Mercury predicted by Johannes Kepler. In 1639, Giovanni Zupi used a telescope to discover that the planet had orbital phases similar to Venus and the Moon. The observation demonstrated conclusively that Mercury orbited around the Sun.[32]
A rare event in astronomy is the passage of one planet in front of another (occultation), as seen from Earth. Mercury and Venus occult each other every few centuries, and the event of May 28, 1737 is the only one historically observed, having been seen by John Bevis at the Royal Greenwich Observatory.[170] The next occultation of Mercury by Venus will be on December 3, 2133.[171]
The difficulties inherent in observing Mercury mean that it was far less studied than the other planets. In 1800, Johann Schröter made observations of surface features, claiming to have observed 20-kilometre-high (12 mi) mountains. Friedrich Bessel used Schröter’s drawings to erroneously estimate the rotation period as 24 hours and an axial tilt of 70°.[172] In the 1880s, Giovanni Schiaparelli mapped the planet more accurately, and suggested that Mercury’s rotational period was 88 days, the same as its orbital period due to tidal locking.[173] This phenomenon is known as synchronous rotation. The effort to map the surface of Mercury was continued by Eugenios Antoniadi, who published a book in 1934 that included both maps and his own observations.[100] Many of the planet’s surface features, particularly the albedo features, take their names from Antoniadi’s map.[174]
In June 1962, Soviet scientists at the Institute of Radio-engineering and Electronics of the USSR Academy of Sciences, led by Vladimir Kotelnikov, became the first to bounce a radar signal off Mercury and receive it, starting radar observations of the planet.[175][176][177] Three years later, radar observations by Americans Gordon H. Pettengill and Rolf B. Dyce, using the 300-meter Arecibo radio telescope in Puerto Rico, showed conclusively that the planet’s rotational period was about 59 days.[178][179] The theory that Mercury’s rotation was synchronous had become widely held, and it was a surprise to astronomers when these radio observations were announced. If Mercury were tidally locked, its dark face would be extremely cold, but measurements of radio emission revealed that it was much hotter than expected. Astronomers were reluctant to drop the synchronous rotation theory and proposed alternative mechanisms such as powerful heat-distributing winds to explain the observations.[180]
Water ice (yellow) at Mercury’s north polar region
Italian astronomer Giuseppe Colombo noted that the rotation value was about two-thirds of Mercury’s orbital period, and proposed that the planet’s orbital and rotational periods were locked into a 3:2 rather than a 1:1 resonance.[181] Data from Mariner 10 subsequently confirmed this view.[182] This means that Schiaparelli’s and Antoniadi’s maps were not «wrong». Instead, the astronomers saw the same features during every second orbit and recorded them, but disregarded those seen in the meantime, when Mercury’s other face was toward the Sun, because the orbital geometry meant that these observations were made under poor viewing conditions.[172]
Ground-based optical observations did not shed much further light on Mercury, but radio astronomers using interferometry at microwave wavelengths, a technique that enables removal of the solar radiation, were able to discern physical and chemical characteristics of the subsurface layers to a depth of several meters.[183][184] Not until the first space probe flew past Mercury did many of its most fundamental morphological properties become known. Moreover, recent technological advances have led to improved ground-based observations. In 2000, high-resolution lucky imaging observations were conducted by the Mount Wilson Observatory 1.5 meter Hale telescope. They provided the first views that resolved surface features on the parts of Mercury that were not imaged in the Mariner 10 mission.[185] Most of the planet has been mapped by the Arecibo radar telescope, with 5 km (3.1 mi) resolution, including polar deposits in shadowed craters of what may be water ice.[186]
Research with space probes
MESSENGER being prepared for launch
Mercury transiting the Sun as viewed by the Mars rover Curiosity (June 3, 2014).[187]
Reaching Mercury from Earth poses significant technical challenges, because it orbits so much closer to the Sun than Earth. A Mercury-bound spacecraft launched from Earth must travel over 91 million kilometres (57 million miles) into the Sun’s gravitational potential well. Mercury has an orbital speed of 47.4 km/s (29.5 mi/s), whereas Earth’s orbital speed is 29.8 km/s (18.5 mi/s).[114] Therefore, the spacecraft must make a large change in velocity (delta-v) to get to Mercury and then enter orbit,[188] as compared to the delta-v required for, say, Mars planetary missions.
The potential energy liberated by moving down the Sun’s potential well becomes kinetic energy, requiring a delta-v change to do anything other than pass by Mercury. Some portion of this delta-v budget can be provided from a gravity assist during one or more fly-bys of Venus.[189] To land safely or enter a stable orbit the spacecraft would rely entirely on rocket motors. Aerobraking is ruled out because Mercury has a negligible atmosphere. A trip to Mercury requires more rocket fuel than that required to escape the Solar System completely. As a result, only three space probes have visited it so far.[190] A proposed alternative approach would use a solar sail to attain a Mercury-synchronous orbit around the Sun.[191]
Mariner 10
Mariner 10, the first probe to visit Mercury
The first spacecraft to visit Mercury was NASA’s Mariner 10 (1974–1975).[26] The spacecraft used the gravity of Venus to adjust its orbital velocity so that it could approach Mercury, making it both the first spacecraft to use this gravitational «slingshot» effect and the first NASA mission to visit multiple planets.[192] Mariner 10 provided the first close-up images of Mercury’s surface, which immediately showed its heavily cratered nature, and revealed many other types of geological features, such as the giant scarps that were later ascribed to the effect of the planet shrinking slightly as its iron core cools.[193] Unfortunately, the same face of the planet was lit at each of Mariner 10‘s close approaches. This made close observation of both sides of the planet impossible,[194] and resulted in the mapping of less than 45% of the planet’s surface.[195]
The spacecraft made three close approaches to Mercury, the closest of which took it to within 327 km (203 mi) of the surface.[196] At the first close approach, instruments detected a magnetic field, to the great surprise of planetary geologists—Mercury’s rotation was expected to be much too slow to generate a significant dynamo effect. The second close approach was primarily used for imaging, but at the third approach, extensive magnetic data were obtained. The data revealed that the planet’s magnetic field is much like Earth’s, which deflects the solar wind around the planet. For many years after the Mariner 10 encounters, the origin of Mercury’s magnetic field remained the subject of several competing theories.[197][198]
On March 24, 1975, just eight days after its final close approach, Mariner 10 ran out of fuel. Because its orbit could no longer be accurately controlled, mission controllers instructed the probe to shut down.[199] Mariner 10 is thought to be still orbiting the Sun, passing close to Mercury every few months.[200]
MESSENGER
Estimated details of the impact of MESSENGER on April 30, 2015
A second NASA mission to Mercury, named MESSENGER (MErcury Surface, Space ENvironment, GEochemistry, and Ranging), was launched on August 3, 2004. It made a fly-by of Earth in August 2005, and of Venus in October 2006 and June 2007 to place it onto the correct trajectory to reach an orbit around Mercury.[201] A first fly-by of Mercury occurred on January 14, 2008, a second on October 6, 2008,[202] and a third on September 29, 2009.[203] Most of the hemisphere not imaged by Mariner 10 was mapped during these fly-bys. The probe successfully entered an elliptical orbit around the planet on March 18, 2011. The first orbital image of Mercury was obtained on March 29, 2011. The probe finished a one-year mapping mission,[202] and then entered a one-year extended mission into 2013. In addition to continued observations and mapping of Mercury, MESSENGER observed the 2012 solar maximum.[204]
The mission was designed to clear up six key issues: Mercury’s high density, its geological history, the nature of its magnetic field, the structure of its core, whether it has ice at its poles, and where its tenuous atmosphere comes from. To this end, the probe carried imaging devices that gathered much-higher-resolution images of much more of Mercury than Mariner 10, assorted spectrometers to determine abundances of elements in the crust, and magnetometers and devices to measure velocities of charged particles. Measurements of changes in the probe’s orbital velocity were expected to be used to infer details of the planet’s interior structure.[205] MESSENGER‘s final maneuver was on April 24, 2015, and it crashed into Mercury’s surface on April 30, 2015.[206][207][208] The spacecraft’s impact with Mercury occurred near 3:26 pm EDT on April 30, 2015, leaving a crater estimated to be 16 m (52 ft) in diameter.[209]
BepiColombo
The European Space Agency and the Japanese Space Agency developed and launched a joint mission called BepiColombo, which will orbit Mercury with two probes: one to map the planet and the other to study its magnetosphere.[210] Launched on October 20, 2018, BepiColombo is expected to reach Mercury in 2025.[211] It will release a magnetometer probe into an elliptical orbit, then chemical rockets will fire to deposit the mapper probe into a circular orbit. Both probes will operate for one terrestrial year.[210] The mapper probe carries an array of spectrometers similar to those on MESSENGER, and will study the planet at many different wavelengths including infrared, ultraviolet, X-ray and gamma ray.[212] BepiColombo conducted the first of its six planned Mercury flybys on October 1, 2021.[213]
See also
- Outline of Mercury (planet)
- Budha, a deity identified with the planet in Hindu astrology
- Colonization of Mercury
- Mercury in astrology
- Mercury in fiction
Notes
- ^ In astronomy, the words «rotation» and «revolution» have different meanings. «Rotation» is the turning of a body about an axis that passes through the body, as in «Earth rotates once a day.» «Revolution» is motion around a centre that is external to the body, usually in orbit, as in «Earth takes a year for each revolution around the Sun.» The verbs «rotate» and «revolve» mean doing rotation and revolution, respectively.
- ^ Pluto was considered a planet from its discovery in 1930 to 2006, but after that it has been reclassified as a dwarf planet. Pluto’s orbital eccentricity is greater than Mercury’s. Pluto is also smaller than Mercury, but was thought to be larger until 1976.
- ^ The Sun’s total angular displacement during its apparent retrograde motion as seen from the surface of Mercury is ~1.23°, while the Sun’s angular diameter when the apparent retrograde motion begins and ends is ~1.71°, increasing to ~1.73° at perihelion (midway through the retrograde motion).
- ^ It is important to be clear about the meaning of «closeness». In the astronomical literature, the term «closest planets» often means «the two planets that approach each other most closely». In other words, the orbits of the two planets approach each other most closely. However, this does not mean that the two planets are closest over time. For example, essentially because Mercury is closer to the Sun than Venus, Mercury spends more time in proximity to Earth; it could, therefore, be said that Mercury is the planet that is «closest to Earth when averaged over time». However, using this time-average definition of ‘closeness’—as noted above—it turns out that Mercury is the closest planet to all other planets in the solar system. For that reason, arguably, the proximity-definition is not particularly helpful. An episode of the BBC Radio 4 programme «More or Less» explains the different notions of proximity well.[19]
- ^ Some sources precede the cuneiform transcription with «MUL». «MUL» is a cuneiform sign that was used in the Sumerian language to designate a star or planet, but it is not considered part of the actual name. The «4» is a reference number in the Sumero–Akkadian transliteration system to designate which of several syllables a certain cuneiform sign is most likely designating.
References
- ^ «Mercurian». Lexico UK English Dictionary. Oxford University Press. Archived from the original on March 27, 2020.
- ^ «Mercurial». Lexico UK English Dictionary UK English Dictionary. Oxford University Press. Archived from the original on December 22, 2019.
- ^ a b c d e f g h i j k l m Williams, David R. (November 25, 2020). «Mercury Fact Sheet». NASA. Retrieved April 19, 2021.
- ^ Souami, D.; Souchay, J. (July 2012). «The solar system’s invariable plane». Astronomy & Astrophysics. 543: 11. Bibcode:2012A&A…543A.133S. doi:10.1051/0004-6361/201219011. A133.
- ^ Yeomans, Donald K. (April 7, 2008). «HORIZONS Web-Interface for Mercury Major Body». JPL Horizons On-Line Ephemeris System. Retrieved April 7, 2008. – Select «Ephemeris Type: Orbital Elements», «Time Span: 2000-01-01 12:00 to 2000-01-02». («Target Body: Mercury» and «Center: Sun» should be defaulted to.) Results are instantaneous osculating values at the precise J2000 epoch.
- ^ a b c d e f g Davis, Phillips; Barnett, Amanda (February 15, 2021). «Mercury». Solar System Exploration. NASA Jet Propulsion Laboratory. Retrieved April 21, 2021.
- ^ Seidelmann, P. Kenneth; Archinal, Brent A.; A’Hearn, Michael F.; et al. (2007). «Report of the IAU/IAG Working Group on cartographic coordinates and rotational elements: 2006». Celestial Mechanics and Dynamical Astronomy. 98 (3): 155–180. Bibcode:2007CeMDA..98..155S. doi:10.1007/s10569-007-9072-y. S2CID 122772353.
- ^ Mazarico, Erwan; Genova, Antonio; Goossens, Sander; Lemoine, Frank G.; Neumann, Gregory A.; Zuber, Maria T.; Smith, David E.; Solomon, Sean C. (2014). «The gravity field, orientation, and ephemeris of Mercury from MESSENGER observations after three years in orbit» (PDF). Journal of Geophysical Research: Planets. 119 (12): 2417–2436. Bibcode:2014JGRE..119.2417M. doi:10.1002/2014JE004675. hdl:1721.1/97927. ISSN 2169-9097. S2CID 42430050.
- ^ a b c Margot, Jean-Luc; Peale, Stanton J.; Solomon, Sean C.; Hauck, Steven A.; Ghigo, Frank D.; Jurgens, Raymond F.; Yseboodt, Marie; Giorgini, Jon D.; Padovan, Sebastiano; Campbell, Donald B. (2012). «Mercury’s moment of inertia from spin and gravity data». Journal of Geophysical Research: Planets. 117 (E12): n/a. Bibcode:2012JGRE..117.0L09M. CiteSeerX 10.1.1.676.5383. doi:10.1029/2012JE004161. ISSN 0148-0227. S2CID 22408219.
- ^ «ESO». ESO. Retrieved June 3, 2021.
- ^ Mallama, Anthony (2017). «The spherical bolometric albedo for planet Mercury». arXiv:1703.02670 [astro-ph.EP].
- ^ Mallama, Anthony; Wang, Dennis; Howard, Russell A. (2002). «Photometry of Mercury from SOHO/LASCO and Earth». Icarus. 155 (2): 253–264. Bibcode:2002Icar..155..253M. doi:10.1006/icar.2001.6723.
- ^ «Atmospheres and Planetary Temperatures». American Chemical Society. July 18, 2013. Retrieved January 3, 2023.
- ^ a b c d Vasavada, Ashwin R.; Paige, David A.; Wood, Stephen E. (February 19, 1999). «Near-Surface Temperatures on Mercury and the Moon and the Stability of Polar Ice Deposits» (PDF). Icarus. 141 (2): 179–193. Bibcode:1999Icar..141..179V. doi:10.1006/icar.1999.6175. Figure 3 with the «TWO model»; Figure 5 for pole.
- ^ a b c Mallama, Anthony; Hilton, James L. (October 2018). «Computing apparent planetary magnitudes for The Astronomical Almanac». Astronomy and Computing. 25: 10–24. arXiv:1808.01973. Bibcode:2018A&C….25…10M. doi:10.1016/j.ascom.2018.08.002. S2CID 69912809.
- ^ a b Milillo, A.; Wurz, P.; Orsini, S.; Delcourt, D.; Kallio, E.; Killen, R. M.; Lammer, H.; Massetti, S.; Mura, A.; Barabash, S.; Cremonese, G.; Daglis, I. A.; Angelis, E.; Lellis, A. M.; Livi, S.; Mangano, V.; Torkar, K. (April 2005). «Surface-Exosphere-Magnetosphere System Of Mercury». Space Science Reviews. 117 (3–4): 397–443. Bibcode:2005SSRv..117..397M. doi:10.1007/s11214-005-3593-z. S2CID 122285073.
- ^ a b Berezhnoy, Alexey A. (January 2018). «Chemistry of impact events on Mercury». Icarus. 300: 210–222. Bibcode:2018Icar..300..210B. doi:10.1016/j.icarus.2017.08.034.
- ^ a b «Venus is not Earth’s closest neighbor». Physics Today. AIP Publishing. March 12, 2019. doi:10.1063/pt.6.3.20190312a. ISSN 1945-0699. S2CID 241077611.
- ^ a b c Harford, Tim (January 11, 2019). «BBC Radio 4 – More or Less, Sugar, Outdoors Play and Planets». BBC.
Oliver Hawkins, more or less alumnus and statistical legend, wrote some code for us, which calculated which planet was closest to the Earth on each day for the past 50 years, and then sent the results to David A. Rothery, professor of planetary geosciences at the Open University.
- ^ Elkins-Tanton, Linda T. (2006). Uranus, Neptune, Pluto, and the Outer Solar System. Infobase Publishing. p. 51. ISBN 978-1-4381-0729-5. Extract of page 51
- ^ «Animated clip of orbit and rotation of Mercury». Sciencenetlinks.com.
- ^ a b Prockter, Louise (2005). Ice in the Solar System (PDF). Vol. 26. Johns Hopkins APL Technical Digest. Retrieved July 27, 2009.
- ^ «NASA Completes MESSENGER Mission with Expected Impact on Mercury’s Surface». Archived from the original on May 3, 2015. Retrieved April 30, 2015.
- ^ «From Mercury orbit, MESSENGER watches a lunar eclipse». Planetary Society. October 10, 2014. Retrieved January 23, 2015.
- ^ «Innovative use of pressurant extends MESSENGER’s Mercury mission». Astronomy.com. December 29, 2014. Retrieved January 22, 2015.
- ^ a b c Dunne, James A.; Burgess, Eric (1978). «Chapter One». The Voyage of Mariner 10 – Mission to Venus and Mercury. NASA History Office.
- ^ Στίλβων, Ἑρμῆς. Liddell, Henry George; Scott, Robert; A Greek–English Lexicon at the Perseus Project.
- ^ «Greek Names of the Planets». April 25, 2010. Retrieved July 14, 2012.
Ermis is the Greek name of the planet Mercury, which is the closest planet to the Sun. It is named after the Greek God of commerce, Ermis or Hermes, who was also the messenger of the Ancient Greek gods.
See also the Greek article about the planet. - ^ Antoniadi, Eugène Michel (1974). The Planet Mercury. Translated from French by Moore, Patrick. Shaldon, Devon: Keith Reid Ltd. pp. 9–11. ISBN 978-0-904094-02-2.
- ^ Duncan, John Charles (1946). Astronomy: A Textbook. Harper & Brothers. p. 125.
The symbol for Mercury represents the Caduceus, a wand with two serpents twined around it, which was carried by the messenger of the gods.
- ^
Jones, Alexander (1999). Astronomical papyri from Oxyrhynchus. pp. 62–63. ISBN 9780871692337.It is now possible to trace the medieval symbols for at least four of the five planets to forms that occur in some of the latest papyrus horoscopes ([ P.Oxy. ] 4272, 4274, 4275 […]). Mercury’s is a stylized caduceus.
- ^ a b c d e f g h Strom, Robert G.; Sprague, Ann L. (2003). Exploring Mercury: the iron planet. Springer. ISBN 978-1-85233-731-5.
- ^ Talbert, Tricia, ed. (March 21, 2012). «MESSENGER Provides New Look at Mercury’s Surprising Core and Landscape Curiosities». NASA.
- ^ «Scientists find evidence Mercury has a solid inner core». AGU Newsroom. Retrieved April 17, 2019.
- ^ «Mercury». US Geological Survey. May 8, 2003. Archived from the original on September 29, 2006. Retrieved November 26, 2006.
- ^ Lyttleton, Raymond A. (1969). «On the Internal Structures of Mercury and Venus». Astrophysics and Space Science. 5 (1): 18–35. Bibcode:1969Ap&SS…5…18L. doi:10.1007/BF00653933. S2CID 122572625.
- ^ Hauck, Steven A.; Margot, Jean-Luc; Solomon, Sean C.; Phillips, Roger J.; Johnson, Catherine L.; Lemoine, Frank G.; Mazarico, Erwan; McCoy, Timothy J.; Padovan, Sebastiano; Peale, Stanton J.; Perry, Mark E.; Smith, David E.; Zuber, Maria T. (2013). «The curious case of Mercury’s internal structure». Journal of Geophysical Research: Planets. 118 (6): 1204–1220. Bibcode:2013JGRE..118.1204H. doi:10.1002/jgre.20091. hdl:1721.1/85633. S2CID 17668886.
- ^ Gold, Lauren (May 3, 2007). «Mercury has molten core, Cornell researcher shows». Chronicle. Cornell University. Retrieved May 12, 2008.
- ^ Finley, Dave (May 3, 2007). «Mercury’s Core Molten, Radar Study Shows». National Radio Astronomy Observatory. Retrieved May 12, 2008.
- ^ a b Spohn, Tilman; Sohl, Frank; Wieczerkowski, Karin; Conzelmann, Vera (2001). «The interior structure of Mercury: what we know, what we expect from BepiColombo». Planetary and Space Science. 49 (14–15): 1561–1570. Bibcode:2001P&SS…49.1561S. doi:10.1016/S0032-0633(01)00093-9.
- ^ Gallant, Roy A. (1986). The National Geographic Picture Atlas of Our Universe (2nd ed.). National Geographic Society. ISBN 9780870446443.
- ^ Padovan, Sebastiano; Wieczorek, Mark A.; Margot, Jean-Luc; Tosi, Nicola; Solomon, Sean C. (2015). «Thickness of the crust of Mercury from geoid-to-topography ratios». Geophysical Research Letters. 42 (4): 1029. Bibcode:2015GeoRL..42.1029P. doi:10.1002/2014GL062487. S2CID 31442257.
- ^ Solomon, Sean C.; Nittler, Larry R.; Anderson, Brian J. (December 20, 2018). Mercury: The View after MESSENGER. Cambridge University Press. p. 534. ISBN 978-1-107-15445-2.
- ^ Sori, Michael M. (May 2018). «A thin, dense crust for Mercury». Earth and Planetary Science Letters. 489: 92–99. Bibcode:2018E&PSL.489…92S. doi:10.1016/j.epsl.2018.02.033.
- ^ Schenk, Paul M.; Melosh, H. Jay (March 1994). «Lobate Thrust Scarps and the Thickness of Mercury’s Lithosphere». Abstracts of the 25th Lunar and Planetary Science Conference. 1994: 1994LPI….25.1203S. Bibcode:1994LPI….25.1203S.
- ^ Watters, T. R.; Nimmo, F.; Robinson, M. S. (2004). Chronology of Lobate Scarp Thrust Faults and the Mechanical Structure of Mercury’s Lithosphere. Lunar and Planetary Science Conference. p. 1886. Bibcode:2004LPI….35.1886W.
- ^ Watters, Thomas R.; Robinson, Mark S.; Cook, Anthony C. (November 1998). «Topography of lobate scarps on Mercury; new constraints on the planet’s contraction». Geology. 26 (11): 991–994. Bibcode:1998Geo….26..991W. doi:10.1130/0091-7613(1998)026<0991:TOLSOM>2.3.CO;2.
- ^ a b c d Benz, W.; Slattery, W. L.; Cameron, Alastair G. W. (1988). «Collisional stripping of Mercury’s mantle». Icarus. 74 (3): 516–528. Bibcode:1988Icar…74..516B. doi:10.1016/0019-1035(88)90118-2.
- ^ a b Cameron, Alastair G. W. (1985). «The partial volatilization of Mercury». Icarus. 64 (2): 285–294. Bibcode:1985Icar…64..285C. doi:10.1016/0019-1035(85)90091-0.
- ^ Weidenschilling, Stuart J. (1987). «Iron/silicate fractionation and the origin of Mercury». Icarus. 35 (1): 99–111. Bibcode:1978Icar…35…99W. doi:10.1016/0019-1035(78)90064-7.
- ^ Sappenfield, Mark (September 29, 2011). «Messenger’s message from Mercury: Time to rewrite the textbooks». The Christian Science Monitor. Retrieved August 21, 2017.
- ^ «BepiColombo». Science & Technology. European Space Agency. Retrieved April 7, 2008.
- ^ Cartwright, Jon (September 30, 2011). «Messenger sheds light on Mercury’s formation». Chemistry World. Retrieved August 21, 2017.
- ^ Morris, Jefferson (November 10, 2008). «Laser Altimetry». Aviation Week & Space Technology. 169 (18): 18.
Mercury’s crust is more analogous to a marbled cake than a layered cake.
- ^ Hughes, E. T.; Vaughan, W. M. (March 2012). Albedo Features of Mercury. 43rd Lunar and Planetary Science Conference, held March 19–23, 2012 at The Woodlands, Texas. Vol. 1659. Bibcode:2012LPI….43.2151H. 2151.
- ^ Blue, Jennifer (April 11, 2008). «Gazetteer of Planetary Nomenclature». US Geological Survey. Retrieved April 11, 2008.
- ^ a b Dunne, James A.; Burgess, Eric (1978). «Chapter Seven». The Voyage of Mariner 10 – Mission to Venus and Mercury. NASA History Office. Retrieved May 28, 2008.
- ^ Nittler, Larry R.; Weider, Shoshana Z. (2019). «The Surface Composition of Mercury». Elements. 15 (1): 33–38. doi:10.2138/gselements.15.1.33. S2CID 135051680.
- ^ «Categories for Naming Features on Planets and Satellites». US Geological Survey. Retrieved August 20, 2011.
- ^ Strom, Robert G. (1979). «Mercury: a post-Mariner assessment». Space Science Reviews. 24 (1): 3–70. Bibcode:1979SSRv…24….3S. doi:10.1007/BF00221842. S2CID 122563809.
- ^ Broadfoot, A. Lyle; Kumar, Shailendra; Belton, Michael J. S.; McElroy, Michael B. (July 12, 1974). «Mercury’s Atmosphere from Mariner 10: Preliminary Results». Science. 185 (4146): 166–169. Bibcode:1974Sci…185..166B. doi:10.1126/science.185.4146.166. PMID 17810510. S2CID 7790470.
- ^ Geology of the solar system. IMAP 2596. U.S. Geological Survey. 1997. doi:10.3133/i2596.
- ^ Head, James W.; Solomon, Sean C. (1981). «Tectonic Evolution of the Terrestrial Planets» (PDF). Science. 213 (4503): 62–76. Bibcode:1981Sci…213…62H. CiteSeerX 10.1.1.715.4402. doi:10.1126/science.213.4503.62. hdl:2060/20020090713. PMID 17741171. Archived from the original (PDF) on July 21, 2018. Retrieved October 25, 2017.
- ^ «Scientists see Mercury in a new light». Science Daily. February 28, 2008. Retrieved April 7, 2008.
- ^ a b c d e f g Spudis, Paul D. (2001). «The Geological History of Mercury». Workshop on Mercury: Space Environment, Surface, and Interior, Chicago (1097): 100. Bibcode:2001mses.conf..100S.
- ^ Ritzel, Rebecca (December 20, 2012). «Ballet isn’t rocket science, but the two aren’t mutually exclusive, either». The Washington Post. Washington, D.C., United States. Retrieved December 22, 2012.
- ^ Shiga, David (January 30, 2008). «Bizarre spider scar found on Mercury’s surface». NewScientist.com news service.
- ^ Schultz, Peter H.; Gault, Donald E. (1975). «Seismic effects from major basin formations on the moon and Mercury». Earth, Moon, and Planets. 12 (2): 159–175. Bibcode:1975Moon…12..159S. doi:10.1007/BF00577875. S2CID 121225801.
- ^ Wieczorek, Mark A.; Zuber, Maria T. (2001). «A Serenitatis origin for the Imbrian grooves and South Pole-Aitken thorium anomaly». Journal of Geophysical Research. 106 (E11): 27853–27864. Bibcode:2001JGR…10627853W. doi:10.1029/2000JE001384. Retrieved May 12, 2008.
- ^ Fassett, Caleb I.; Head, James W.; Baker, David M. H.; Zuber, Maria T.; Smith, David E.; Neumann, Gregory A.; Solomon, Sean C.; Klimczak, Christian; Strom, Robert G.; Chapman, Clark R.; Prockter, Louise M.; Phillips, Roger J.; Oberst, Jürgen; Preusker, Frank (October 2012). «Large impact basins on Mercury: Global distribution, characteristics, and modification history from MESSENGER orbital data». Journal of Geophysical Research. 117. 15 pp. Bibcode:2012JGRE..117.0L08F. doi:10.1029/2012JE004154. E00L08.
- ^ Denevi, Brett W.; Robinson, Mark S. (2008). «Albedo of Immature Mercurian Crustal Materials: Evidence for the Presence of Ferrous Iron». Lunar and Planetary Science. 39 (1391): 1750. Bibcode:2008LPI….39.1750D.
- ^ a b Wagner, Roland J.; Wolf, Ursula; Ivanov, Boris A.; Neukum, Gerhard (October 4–5, 2001). Application of an Updated Impact Cratering Chronology Model to Mercury’ s Time-Stratigraphic System. Workshop on Mercury: Space Environment, Surface, and Interior. Proceedings of a workshop held at The Field Museum. Chicago, IL: Lunar and Planetary Science Institute. p. 106. Bibcode:2001mses.conf..106W.
- ^ Schleicher, Lisa S.; Watters, Thomas R.; Martin, Aaron J.; Banks, Maria E. (October 2019). «Wrinkle ridges on Mercury and the Moon within and outside of mascons». Icarus. 331: 226–237. Bibcode:2019Icar..331..226S. doi:10.1016/j.icarus.2019.04.013. S2CID 150072193.
- ^ a b Choi, Charles Q. (September 26, 2016). «Mercuryquakes May Currently Shake Up the Tiny Planet». Space.com. Retrieved September 28, 2016.
- ^ Dzurisin, Daniel (October 10, 1978). «The tectonic and volcanic history of Mercury as inferred from studies of scarps, ridges, troughs, and other lineaments». Journal of Geophysical Research. 83 (B10): 4883–4906. Bibcode:1978JGR….83.4883D. doi:10.1029/JB083iB10p04883.
- ^ a b Watters, Thomas R.; Daud, Katie; Banks, Maria E.; Selvans, Michelle M.; Chapman, Clark R.; Ernst, Carolyn M. (September 26, 2016). «Recent tectonic activity on Mercury revealed by small thrust fault scarps». Nature Geoscience. 9 (10): 743–747. Bibcode:2016NatGe…9..743W. doi:10.1038/ngeo2814.
- ^ Giacomini, L.; Massironi, M.; Galluzzi, V.; Ferrari, S.; Palumbo, P. (May 2020). «Dating long thrust systems on Mercury: New clues on the thermal evolution of the planet». Geoscience Frontiers. 11 (3): 855–870. doi:10.1016/j.gsf.2019.09.005. S2CID 210298205.
- ^ Kerber, Laura; Head, James W.; Solomon, Sean C.; Murchie, Scott L.; Blewett, David T. (August 15, 2009). «Explosive volcanic eruptions on Mercury: Eruption conditions, magma volatile content, and implications for interior volatile abundances». Earth and Planetary Science Letters. 285 (3–4): 263–271. Bibcode:2009E&PSL.285..263K. doi:10.1016/j.epsl.2009.04.037.
- ^ Head, James W.; Chapman, Clark R.; Strom, Robert G.; Fassett, Caleb I.; Denevi, Brett W. (September 30, 2011). «Flood Volcanism in the Northern High Latitudes of Mercury Revealed by MESSENGER» (PDF). Science. 333 (6051): 1853–1856. Bibcode:2011Sci…333.1853H. doi:10.1126/science.1211997. PMID 21960625. S2CID 7651992.
- ^ Thomas, Rebecca J.; Rothery, David A.; Conway, Susan J.; Anand, Mahesh (September 16, 2014). «Long-lived explosive volcanism on Mercury». Geophysical Research Letters. 41 (17): 6084–6092. Bibcode:2014GeoRL..41.6084T. doi:10.1002/2014GL061224. S2CID 54683272.
- ^ a b c Groudge, Timothy A.; Head, James W. (March 2014). «Global inventory and characterization of pyroclastic deposits on Mercury: New insights into pyroclastic activity from MESSENGER orbital data» (PDF). Journal of Geophysical Research. 119 (3): 635–658. Bibcode:2014JGRE..119..635G. doi:10.1002/2013JE004480. S2CID 14393394.
- ^ a b c Rothery, David A.; Thomas, Rebeca J.; Kerber, Laura (January 1, 2014). «Prolonged eruptive history of a compound volcano on Mercury: Volcanic and tectonic implications» (PDF). Earth and Planetary Science Letters. 385: 59–67. Bibcode:2014E&PSL.385…59R. doi:10.1016/j.epsl.2013.10.023.
- ^ a b Chang, Kenneth (November 29, 2012). «On Closest Planet to the Sun, NASA Finds Lots of Ice». The New York Times. p. A3. Archived from the original on November 29, 2012.
Sean C. Solomon, the principal investigator for MESSENGER, said there was enough ice there to encase Washington, D.C., in a frozen block two and a half miles deep.
- ^ Lewis, John S. (2004). Physics and Chemistry of the Solar System (2nd ed.). Academic Press. p. 463. ISBN 978-0-12-446744-6.
- ^ Murdock, Thomas L.; Ney, Edward P. (1970). «Mercury: The Dark-Side Temperature». Science. 170 (3957): 535–537. Bibcode:1970Sci…170..535M. doi:10.1126/science.170.3957.535. PMID 17799708. S2CID 38824994.
- ^ Lewis, John S. (2004). Physics and Chemistry of the Solar System. Academic Press. ISBN 978-0-12-446744-6. Retrieved June 3, 2008.
- ^ Ingersoll, Andrew P.; Svitek, Tomas; Murray, Bruce C. (1992). «Stability of polar frosts in spherical bowl-shaped craters on the Moon, Mercury, and Mars». Icarus. 100 (1): 40–47. Bibcode:1992Icar..100…40I. doi:10.1016/0019-1035(92)90016-Z.
- ^ Slade, Martin A.; Butler, Bryan J.; Muhleman, Duane O. (1992). «Mercury radar imaging – Evidence for polar ice». Science. 258 (5082): 635–640. Bibcode:1992Sci…258..635S. doi:10.1126/science.258.5082.635. PMID 17748898. S2CID 34009087.
- ^ Williams, David R. (June 2, 2005). «Ice on Mercury». NASA Goddard Space Flight Center. Retrieved May 23, 2008.
- ^ a b c Rawlins, Katherine; Moses, Julianne I.; Zahnle, Kevin J. (1995). «Exogenic Sources of Water for Mercury’s Polar Ice». Bulletin of the American Astronomical Society. 27: 1117. Bibcode:1995DPS….27.2112R.
- ^ Harmon, John K.; Perillat, Phil J.; Slade, Martin A. (2001). «High-Resolution Radar Imaging of Mercury’s North Pole». Icarus. 149 (1): 1–15. Bibcode:2001Icar..149….1H. doi:10.1006/icar.2000.6544.
- ^ Domingue DL, Koehn PL, et al. (2009). «Mercury’s Atmosphere: A Surface-Bounded Exosphere». Space Science Reviews. 131 (1–4): 161–186. Bibcode:2007SSRv..131..161D. doi:10.1007/s11214-007-9260-9. S2CID 121301247.
- ^ Hunten, Donald M.; Shemansky, Donald Eugene; Morgan, Thomas Hunt (1988). «The Mercury atmosphere». In Vilas, Faith; Chapman, Clark R.; Shapley Matthews, Mildred (eds.). Mercury. University of Arizona Press. ISBN 978-0-8165-1085-6.
- ^ Lakdawalla, Emily (July 3, 2008). «MESSENGER Scientists «Astonished» to Find Water in Mercury’s Thin Atmosphere». The Planetary Society. Retrieved May 18, 2009.
- ^ Zurbuchen TH, Raines JM, et al. (2008). «MESSENGER Observations of the Composition of Mercury’s Ionized Exosphere and Plasma Environment». Science. 321 (5885): 90–92. Bibcode:2008Sci…321…90Z. doi:10.1126/science.1159314. PMID 18599777. S2CID 206513512.
- ^ «Instrument Shows What Planet Mercury Is Made Of». University of Michigan. June 30, 2008. Retrieved May 18, 2009.
- ^ Killen, Rosemary; Cremonese, Gabrielle; et al. (2007). «Processes that Promote and Deplete the Exosphere of Mercury». Space Science Reviews. 132 (2–4): 433–509. Bibcode:2007SSRv..132..433K. doi:10.1007/s11214-007-9232-0. S2CID 121944553.
- ^ Killen, Rosemary M.; Hahn, Joseph M. (December 10, 2014). «Impact Vaporization as a Possible Source of Mercury’s Calcium Exosphere». Icarus. 250: 230–237. Bibcode:2015Icar..250..230K. doi:10.1016/j.icarus.2014.11.035. hdl:2060/20150010116.
- ^ McClintock, William E.; Vervack, Ronald J.; et al. (2009). «MESSENGER Observations of Mercury’s Exosphere: Detection of Magnesium and Distribution of Constituents». Science. 324 (5927): 610–613. Bibcode:2009Sci…324..610M. doi:10.1126/science.1172525. PMID 19407195. S2CID 5578520.
- ^ a b c d e Beatty, J. Kelly; Petersen, Carolyn Collins; Chaikin, Andrew (1999). The New Solar System. Cambridge University Press. ISBN 978-0-521-64587-4.
- ^ «Mercury». NASA. October 19, 2021. Retrieved July 4, 2022.
- ^ Hall, Shannon (March 24, 2020). «Life on the Planet Mercury? ‘It’s Not Completely Nuts’ – A new explanation for the rocky world’s jumbled landscape opens a possibility that it could have had ingredients for habitability». The New York Times. Archived from the original on March 24, 2020. Retrieved March 26, 2020.
- ^ Rodriguez, J. Alexis P.; Leonard, Gregory J.; Kargel, Jeffrey S.; Domingue, Deborah; Berman, Daniel C.; Banks, Maria; Zarroca, Mario; Linares, Rogelio; Marchi, Simone; Baker, Victor R.; Webster, Kevin D.; Sykes, Mark (March 16, 2020). «The Chaotic Terrains of Mercury Reveal a History of Planetary Volatile Retention and Loss in the Innermost Solar System». Scientific Reports. 10 (4737): 4737. Bibcode:2020NatSR..10.4737R. doi:10.1038/s41598-020-59885-5. PMC 7075900. PMID 32179758.
- ^ «Vast Collapsed Terrains on Mercury Might be Windows Into Ancient – Possibly Habitable – Volatile-Rich Materials». Planetary Science Institute. March 16, 2020. Retrieved August 27, 2022.
- ^ Seeds, Michael A. (2004). Astronomy: The Solar System and Beyond (4th ed.). Brooks Cole. ISBN 978-0-534-42111-3.
- ^ Williams, David R. (January 6, 2005). «Planetary Fact Sheets». NASA National Space Science Data Center. Retrieved August 10, 2006.
- ^ a b c «Mercury’s Internal Magnetic Field». NASA. January 30, 2008. Retrieved April 21, 2021.
- ^ Gold, Lauren (May 3, 2007). «Mercury has molten core, Cornell researcher shows». Cornell University. Retrieved April 7, 2008.
- ^ Christensen, Ulrich R. (2006). «A deep dynamo generating Mercury’s magnetic field». Nature. 444 (7122): 1056–1058. Bibcode:2006Natur.444.1056C. doi:10.1038/nature05342. PMID 17183319. S2CID 4342216.
- ^ Padovan, Sebastiano; Margot, Jean-Luc; Hauck, Steven A.; Moore, William B.; Solomon, Sean C. (April 2014). «The tides of Mercury and possible implications for its interior structure». Journal of Geophysical Research: Planets. 119 (4): 850–866. Bibcode:2014JGRE..119..850P. doi:10.1002/2013JE004459. S2CID 56282397.
- ^ a b Steigerwald, Bill (June 2, 2009). «Magnetic Tornadoes Could Liberate Mercury’s Tenuous Atmosphere». NASA Goddard Space Flight Center. Retrieved July 18, 2009.
- ^ Van Hoolst, Tim; Jacobs, Carla (2003). «Mercury’s tides and interior structure». Journal of Geophysical Research. 108 (E11): 7. Bibcode:2003JGRE..108.5121V. doi:10.1029/2003JE002126.
- ^ «Space Topics: Compare the Planets: Mercury, Venus, Earth, The Moon, and Mars». Planetary Society. Archived from the original on July 28, 2011. Retrieved April 12, 2007.
- ^ a b Williams, David R. (October 21, 2019). «Planetary Fact Sheet – Metric». NASA. Retrieved April 20, 2021.
- ^ Espenak, Fred (April 21, 2005). «Transits of Mercury». NASA/Goddard Space Flight Center. Retrieved May 20, 2008.
- ^ Biswas, Sukumar (2000). Cosmic Perspectives in Space Physics. Astrophysics and Space Science Library. Springer. p. 176. ISBN 978-0-7923-5813-8.
- ^ a b Margot, J. L.; Peale, S. J.; Jurgens, R. F.; Slade, M. A.; et al. (2007). «Large Longitude Libration of Mercury Reveals a Molten Core». Science. 316 (5825): 710–714. Bibcode:2007Sci…316..710M. doi:10.1126/science.1140514. PMID 17478713. S2CID 8863681.
- ^ Popular Astronomy: A Review of Astronomy and Allied Sciences. Goodsell Observatory of Carleton College. 1896.
although in the case of Venus the libration in longitude due to the eccentricity of the orbit amounts to only 47′ on either side of the mean position, in the case of Mercury it amounts to 23° 39′
- ^ Seligman, C. «The Rotation of Mercury». cseligman.com. NASA Flash animation. Retrieved July 31, 2019.
- ^ van Hemerlrijck, E. (August 1983). «On the Variations in the Insolation at Mercury Resulting from Oscillations of the Orbital Eccentricity». The Moon and the Planets. 29 (1): 83–93. Bibcode:1983M&P….29…83V. doi:10.1007/BF00928377. S2CID 122761699.
- ^ Mercury Closest Approaches to Earth generated with:
1. Solex 10 Archived December 20, 2008, at the Wayback Machine (Text Output file Archived March 9, 2012, at the Wayback Machine)
2. Gravity Simulator charts Archived September 12, 2014, at the Wayback Machine
3. JPL Horizons 1950–2200 Archived November 6, 2015, at the Wayback Machine(3 sources are provided to address original research concerns and to support general long-term trends)
- ^ Stockman, Tom; Monroe, Gabriel; Cordner, Samuel (March 12, 2019). «Venus is not Earth’s closest neighbor». Physics Today. doi:10.1063/PT.6.3.20190312a. S2CID 241077611.
- ^ Stockman, Tom (March 7, 2019). Mercury is the closest planet to all seven other planets (video). Archived from the original on October 28, 2021. Retrieved May 29, 2019 – via YouTube.
- ^ 🌍 Which Planet is the Closest?, archived from the original on October 28, 2021, retrieved July 22, 2021
- ^ Davies, M. E. (June 10, 1975). «Surface Coordinates and Cartography of Mercury». Journal of Geophysical Research. 80 (B17): 2417–2430. Bibcode:1975JGR….80.2417D. doi:10.1029/JB080i017p02417.
- ^ Davies, M. E.; Dwornik, S. E.; Gault, D. E.; Strom, R. G. (1978). NASA Atlas of Mercury. NASA Scientific and Technical Information Office.
- ^ «USGS Astrogeology: Rotation and pole position for the Sun and planets (IAU WGCCRE)». Archived from the original on October 24, 2011. Retrieved October 22, 2009.
- ^ Archinal, Brent A.; A’Hearn, Michael F.; Bowell, Edward L.; Conrad, Albert R.; et al. (2010). «Report of the IAU Working Group on Cartographic Coordinates and Rotational Elements: 2009». Celestial Mechanics and Dynamical Astronomy. 109 (2): 101–135. Bibcode:2011CeMDA.109..101A. doi:10.1007/s10569-010-9320-4. ISSN 0923-2958. S2CID 189842666.
- ^ Liu, Han-Shou; O’Keefe, John A. (1965). «Theory of Rotation for the Planet Mercury». Science. 150 (3704): 1717. Bibcode:1965Sci…150.1717L. doi:10.1126/science.150.3704.1717. PMID 17768871. S2CID 45608770.
- ^ a b Colombo, Giuseppe; Shapiro, Irwin I. (1966). «The rotation of the planet Mercury». Astrophysical Journal. 145: 296. Bibcode:1966ApJ…145..296C. doi:10.1086/148762.
- ^ Correia, Alexandre C. M.; Laskar, Jacques (2009). «Mercury’s capture into the 3/2 spin-orbit resonance including the effect of core–mantle friction». Icarus. 201 (1): 1–11. arXiv:0901.1843. Bibcode:2009Icar..201….1C. doi:10.1016/j.icarus.2008.12.034. S2CID 14778204.
- ^ Correia, Alexandre C. M.; Laskar, Jacques (2004). «Mercury’s capture into the 3/2 spin-orbit resonance as a result of its chaotic dynamics». Nature. 429 (6994): 848–850. Bibcode:2004Natur.429..848C. doi:10.1038/nature02609. PMID 15215857. S2CID 9289925.
- ^ Noyelles, B.; Frouard, J.; Makarov, V. V. & Efroimsky, M. (2014). «Spin-orbit evolution of Mercury revisited». Icarus. 241 (2014): 26–44. arXiv:1307.0136. Bibcode:2014Icar..241…26N. doi:10.1016/j.icarus.2014.05.045. S2CID 53690707.
- ^ Laskar, Jacques (March 18, 2008). «Chaotic diffusion in the Solar System». Icarus. 196 (1): 1–15. arXiv:0802.3371. Bibcode:2008Icar..196….1L. doi:10.1016/j.icarus.2008.02.017. S2CID 11586168.
- ^ Laskar, Jacques; Gastineau, Mickaël (June 11, 2009). «Existence of collisional trajectories of Mercury, Mars and Venus with the Earth». Nature. 459 (7248): 817–819. Bibcode:2009Natur.459..817L. doi:10.1038/nature08096. PMID 19516336. S2CID 4416436.
- ^ Le Verrier, Urbain (1859). «Lettre de M. Le Verrier à M. Faye sur la théorie de Mercure et sur le mouvement du périhélie de cette planète». Comptes rendus hebdomadaires des séances de l’Académie des sciences (in French). Paris. 49: 379–383. (At p. 383 in the same volume Le Verrier’s report is followed by another, from Faye, enthusiastically recommending to astronomers to search for a previously undetected intra-mercurial object.)
- ^ Baum, Richard; Sheehan, William (1997). In Search of Planet Vulcan, The Ghost in Newton’s Clockwork Machine. New York: Plenum Press. ISBN 978-0-306-45567-4.
- ^ a b Clemence, Gerald M. (1947). «The Relativity Effect in Planetary Motions». Reviews of Modern Physics. 19 (4): 361–364. Bibcode:1947RvMP…19..361C. doi:10.1103/RevModPhys.19.361.
- ^ Gilvarry, John J. (1953). «Relativity Precession of the Asteroid Icarus». Physical Review. 89 (5): 1046. Bibcode:1953PhRv…89.1046G. doi:10.1103/PhysRev.89.1046.
- ^ Brown, Kevin. «6.2 Anomalous Precession». Reflections on Relativity. MathPages. Retrieved May 22, 2008.
- ^ a b Menzel, Donald H. (1964). A Field Guide to the Stars and Planets. The Peterson Field Guide Series. Boston: Houghton Mifflin Co. pp. 292–293.
- ^ Tezel, Tunç (January 22, 2003). «Total Solar Eclipse of 2006 March 29». Department of Physics at Fizik Bolumu in Turkey. Retrieved May 24, 2008.
- ^ a b Mallama, Anthony (2011). «Planetary magnitudes». Sky and Telescope. 121 (1): 51–56.
- ^ Espenak, Fred (1996). «NASA Reference Publication 1349; Venus: Twelve year planetary ephemeris, 1995–2006». Twelve Year Planetary Ephemeris Directory. NASA. Retrieved May 24, 2008.
- ^ a b Walker, John. «Mercury Chaser’s Calculator». Fourmilab Switzerland. Retrieved May 29, 2008. (look at 1964 and 2013)
- ^ «Mercury Elongation and Distance». Archived from the original on May 11, 2013. Retrieved May 30, 2008. – Numbers generated using the Solar System Dynamics Group, Horizons On-Line Ephemeris System
- ^ a b c Kelly, Patrick, ed. (2007). Observer’s Handbook 2007. Royal Astronomical Society of Canada. ISBN 978-0-9738109-3-6.
- ^ Curtis, A. C. (October 1972). «Finding Venus or Mercury in daylight». Journal of the British Astronomical Association. 82: 438–439. Bibcode:1972JBAA…82..438C.
- ^ Alers, Paul E. (March 17, 2011). «Celebrating Mercury Orbit». NASA Multimedia. Retrieved March 18, 2011.
- ^ «NASA spacecraft now circling Mercury – a first». NBC News. March 17, 2011. Retrieved March 24, 2011.
- ^ Baumgardner, Jeffrey; Mendillo, Michael; Wilson, Jody K. (2000). «A Digital High-Definition Imaging System for Spectral Studies of Extended Planetary Atmospheres. I. Initial Results in White Light Showing Features on the Hemisphere of Mercury Unimaged by Mariner 10». The Astronomical Journal. 119 (5): 2458–2464. Bibcode:2000AJ….119.2458B. doi:10.1086/301323. S2CID 17361371.
- ^ Schaefer, Bradley E. (2007). «The Latitude and Epoch for the Origin of the Astronomical Lore in MUL.APIN». American Astronomical Society Meeting 210, #42.05. 38: 157. Bibcode:2007AAS…210.4205S.
- ^ Hunger, Hermann; Pingree, David (1989). «MUL.APIN: An Astronomical Compendium in Cuneiform». Archiv für Orientforschung. 24: 146.
- ^ «MESSENGER: Mercury and Ancient Cultures». NASA JPL. 2008. Retrieved April 7, 2008.
- ^ Heath, Sir Thomas (1921). A History of Greek Mathematics. Vol. II. Oxford: Clarendon Press. pp. vii, 273.
- ^ Goldstein, Bernard R. (1996). «The Pre-telescopic Treatment of the Phases and Apparent Size of Venus». Journal for the History of Astronomy. 27: 1. Bibcode:1996JHA….27….1G. doi:10.1177/002182869602700101. S2CID 117218196.
- ^ Kelley, David H.; Milone, E. F.; Aveni, Anthony F. (2004). Exploring Ancient Skies: An Encyclopedic Survey of Archaeoastronomy. Birkhäuser. ISBN 978-0-387-95310-6.
- ^ De Groot, Jan Jakob Maria (1912). Religion in China: universism. a key to the study of Taoism and Confucianism. American lectures on the history of religions. Vol. 10. G. P. Putnam’s Sons. p. 300. Retrieved January 8, 2010.
- ^ Crump, Thomas (1992). The Japanese numbers game: the use and understanding of numbers in modern Japan. Nissan Institute/Routledge Japanese studies series. Routledge. pp. 39–40. ISBN 978-0-415-05609-0.
- ^ Hulbert, Homer Bezaleel (1909). The passing of Korea. Doubleday, Page & company. p. 426. Retrieved January 8, 2010.
- ^ Pujari, R. M.; Kolhe, Pradeep; Kumar, N. R. (2006). Pride of India: A Glimpse Into India’s Scientific Heritage. Samskrita Bharati. ISBN 978-81-87276-27-2.
- ^ Bakich, Michael E. (2000). The Cambridge Planetary Handbook. Cambridge University Press. ISBN 978-0-521-63280-5.
- ^ Milbrath, Susan (1999). Star Gods of the Maya: Astronomy in Art, Folklore and Calendars. University of Texas Press. ISBN 978-0-292-75226-9.
- ^ Samsó, Julio; Mielgo, Honorino (1994). «Ibn al-Zarqālluh on Mercury». Journal for the History of Astronomy. 25 (4): 289–96 [292]. Bibcode:1994JHA….25..289S. doi:10.1177/002182869402500403. S2CID 118108131.
- ^ Hartner, Willy (1955). «The Mercury Horoscope of Marcantonio Michiel of Venice». Vistas in Astronomy. 1 (1): 84–138. Bibcode:1955VA……1…84H. doi:10.1016/0083-6656(55)90016-7. at pp. 118–122.
- ^ Ansari, S. M. Razaullah (2002). History of oriental astronomy: proceedings of the joint discussion-17 at the 23rd General Assembly of the International Astronomical Union, organised by the Commission 41 (History of Astronomy), held in Kyoto, August 25–26, 1997. Springer Science+Business Media. p. 137. ISBN 1-4020-0657-8.
- ^ Goldstein, Bernard R. (1969). «Some Medieval Reports of Venus and Mercury Transits». Centaurus. 14 (1): 49–59. Bibcode:1969Cent…14…49G. doi:10.1111/j.1600-0498.1969.tb00135.x.
- ^ Ramasubramanian, K.; Srinivas, M. S.; Sriram, M. S. (1994). «Modification of the Earlier Indian Planetary Theory by the Kerala Astronomers (c. 1500 AD) and the Implied Heliocentric Picture of Planetary Motion» (PDF). Current Science. 66: 784–790. Archived from the original (PDF) on December 23, 2010. Retrieved April 23, 2010.
- ^ Gaab, Hans (2018). Simon Marius and His Research. Springer. p. 256. ISBN 978-3-319-92620-9.
Marius noted in the dedication from June 30, 1612, in the Prognosticon auf 1613 «that Mercury is illuminated by the Sun in the same way as the Venus and the Moon» and reports his observations of the brightness.
- ^ Sinnott, Roger W.; Meeus, Jean (1986). «John Bevis and a Rare Occultation». Sky and Telescope. 72: 220. Bibcode:1986S&T….72..220S.
- ^ Ferris, Timothy (2003). Seeing in the Dark: How Amateur Astronomers. Simon and Schuster. ISBN 978-0-684-86580-5.
- ^ a b Colombo, Giuseppe; Shapiro, Irwin I. (November 1965). «The Rotation of the Planet Mercury». SAO Special Report #188R. 188: 188. Bibcode:1965SAOSR.188…..C.
- ^ Holden, Edward S. (1890). «Announcement of the Discovery of the Rotation Period of Mercury [by Professor Schiaparelli]». Publications of the Astronomical Society of the Pacific. 2 (7): 79. Bibcode:1890PASP….2…79H. doi:10.1086/120099. S2CID 122095054.
- ^ Davies, Merton E.; Dwornik, Stephen E.; Gault, Donald E.; Strom, Robert G. (1978). «Surface Mapping». Atlas of Mercury. NASA Office of Space Sciences. Retrieved May 28, 2008.
- ^ Evans, John V.; Brockelman, Richard A.; Henry, John C.; Hyde, Gerald M.; Kraft, Leon G.; Reid, Wyatt A.; Smith, W. W. (1965). «Radio Echo Observations of Venus and Mercury at 23 cm Wavelength». Astronomical Journal. 70: 487–500. Bibcode:1965AJ…..70..486E. doi:10.1086/109772.
- ^ Moore, Patrick (2000). The Data Book of Astronomy. New York: CRC Press. p. 483. ISBN 978-0-7503-0620-1.
- ^ Butrica, Andrew J. (1996). «Chapter 5». To See the Unseen: A History of Planetary Radar Astronomy. NASA History Office, Washington D.C. ISBN 978-0-16-048578-7.
- ^ Pettengill, Gordon H.; Dyce, Rolf B. (1965). «A Radar Determination of the Rotation of the Planet Mercury». Nature. 206 (1240): 451–2. Bibcode:1965Natur.206Q1240P. doi:10.1038/2061240a0. S2CID 31525579.
- ^ «Mercury». Eric Weisstein’s World of Astronomy. Wolfram Research. Retrieved April 18, 2021.
- ^ Murray, Bruce C.; Burgess, Eric (1977). Flight to Mercury. Columbia University Press. ISBN 978-0-231-03996-3.
- ^ Colombo, Giuseppe (1965). «Rotational Period of the Planet Mercury». Nature. 208 (5010): 575. Bibcode:1965Natur.208..575C. doi:10.1038/208575a0. S2CID 4213296.
- ^ Davies, Merton E.; et al. (1976). «Mariner 10 Mission and Spacecraft». SP-423 Atlas of Mercury. NASA JPL. Retrieved April 7, 2008.
- ^ Golden, Leslie M. (1977). A Microwave Interferometric Study of the Subsurface of the Planet Mercury (Thesis). University of California, Berkeley. Bibcode:1977PhDT………9G.
- ^ Mitchell, David L.; De Pater, Imke (1994). «Microwave Imaging of Mercury’s Thermal Emission at Wavelengths from 0.3 to 20.5 cm (1994)». Icarus. 110 (1): 2–32. Bibcode:1994Icar..110….2M. doi:10.1006/icar.1994.1105.
- ^ Dantowitz, Ronald F.; Teare, Scott W.; Kozubal, Marek J. (2000). «Ground-based High-Resolution Imaging of Mercury». Astronomical Journal. 119 (4): 2455–2457. Bibcode:2000AJ….119.2455D. doi:10.1086/301328. S2CID 121483006.
- ^ Harmon, John K.; Slade, Martin A.; Butler, Bryan J.; Head III, James W.; Rice, Melissa S.; Campbell, Donald B. (2007). «Mercury: Radar images of the equatorial and midlatitude zones». Icarus. 187 (2): 374–405. Bibcode:2007Icar..187..374H. doi:10.1016/j.icarus.2006.09.026.
- ^ Webster, Guy (June 10, 2014). «Mercury Passes in Front of the Sun, as Seen From Mars». NASA. Retrieved June 10, 2014.
- ^ Zacny, Kris (July 2, 2015). Inner Solar System: Prospective Energy and Material Resources. Springer International Publishing. p. 154. ISBN 9783319195698.
- ^ Wagner, Sam; Wie, Bong (November 2015). «Hybrid Algorithm for Multiple Gravity-Assist and Impulsive Delta-V Maneuvers». Journal of Guidance, Control, and Dynamics. 38 (11): 2096–2107. Bibcode:2015JGCD…38.2096W. doi:10.2514/1.G000874.
- ^ «Mercury» (PDF). NASA Jet Propulsion Laboratory. May 5, 2008. Retrieved April 26, 2021.
- ^ Leipold, Manfred E.; Seboldt, W.; Lingner, Stephan; Borg, Erik; Herrmann, Axel Siegfried; Pabsch, Arno; Wagner, O.; Brückner, Johannes (1996). «Mercury sun-synchronous polar orbiter with a solar sail». Acta Astronautica. 39 (1): 143–151. Bibcode:1996AcAau..39..143L. doi:10.1016/S0094-5765(96)00131-2.
- ^ Dunne, James A. & Burgess, Eric (1978). «Chapter Four». The Voyage of Mariner 10 – Mission to Venus and Mercury. NASA History Office. Retrieved May 28, 2008.
- ^ Phillips, Tony (October 1976). «NASA 2006 Transit of Mercury». SP-423 Atlas of Mercury. NASA. Retrieved April 7, 2008.
- ^ «BepiColumbo – Background Science». European Space Agency. Retrieved June 18, 2017.
- ^ Malik, Tariq (August 16, 2004). «MESSENGER to test theory of shrinking Mercury». USA Today. Retrieved May 23, 2008.
- ^ Davies ME, et al. (1978). «Mariner 10 Mission and Spacecraft». Atlas of Mercury. NASA Office of Space Sciences. Retrieved May 30, 2008.
- ^ Ness, Norman F. (1978). «Mercury – Magnetic field and interior». Space Science Reviews. 21 (5): 527–553. Bibcode:1978SSRv…21..527N. doi:10.1007/BF00240907. S2CID 120025983.
- ^ Aharonson, Oded; Zuber, Maria T; Solomon, Sean C (2004). «Crustal remanence in an internally magnetized non-uniform shell: a possible source for Mercury’s magnetic field?». Earth and Planetary Science Letters. 218 (3–4): 261–268. Bibcode:2004E&PSL.218..261A. doi:10.1016/S0012-821X(03)00682-4.
- ^ Dunne, James A. & Burgess, Eric (1978). «Chapter Eight». The Voyage of Mariner 10 – Mission to Venus and Mercury. NASA History Office.
- ^ Grayzeck, Ed (April 2, 2008). «Mariner 10». NSSDC Master Catalog. NASA. Retrieved April 7, 2008.
- ^ «MESSENGER Engine Burn Puts Spacecraft on Track for Venus». SpaceRef.com. 2005. Retrieved March 2, 2006.
- ^ a b «Countdown to MESSENGER’s Closest Approach with Mercury». Johns Hopkins University Applied Physics Laboratory. January 14, 2008. Archived from the original on May 13, 2013. Retrieved May 30, 2008.
- ^ «MESSENGER Gains Critical Gravity Assist for Mercury Orbital Observations». MESSENGER Mission News. September 30, 2009. Archived from the original on May 10, 2013. Retrieved September 30, 2009.
- ^ «NASA extends spacecraft’s Mercury mission». United Press International. November 15, 2011. Retrieved November 16, 2011.
- ^ «MESSENGER: Fact Sheet» (PDF). Applied Physics Laboratory. February 2011. Retrieved August 21, 2017.
- ^ Wall, Mike (March 29, 2015). «NASA Mercury Probe Trying to Survive for Another Month». Space.com. Retrieved April 4, 2015.
- ^ Chang, Kenneth (April 27, 2015). «NASA’s Messenger Mission Is Set to Crash Into Mercury». The New York Times. Archived from the original on April 29, 2015. Retrieved April 27, 2015.
- ^ Corum, Jonathan (April 30, 2015). «Messenger’s Collision Course With Mercury». The New York Times. Retrieved April 30, 2015.
- ^ «Best Determination of MESSENGER’s Impact Location». MESSENGER Featured Images. Johns Hopkins – Applied Physics Lab. June 3, 2015. Retrieved April 29, 2015.
- ^ a b «ESA gives go-ahead to build BepiColombo». European Space Agency. February 26, 2007. Retrieved May 29, 2008.
- ^ «BepiColombo Fact Sheet». European Space Agency. December 1, 2016. Retrieved December 19, 2016.
- ^ «Objectives». European Space Agency. February 21, 2006. Retrieved May 29, 2008.
- ^ Warren, Haygen (October 24, 2021). «BepiColombo completes first Mercury flyby, science provides insight into planet’s unique environment». NASA Spaceflight. Retrieved October 8, 2022.
External links
This audio file was created from a revision of this article dated 16 January 2008, and does not reflect subsequent edits.
- Atlas of Mercury. NASA. 1978. SP-423.
- Mercury nomenclature and map with feature names from the USGS/IAU Gazetteer of Planetary Nomenclature
- Equirectangular map of Mercury by Applied Coherent Technology Corp
- 3D globe of Mercury by Google
- Mercury at Solarviews.com
- Mercury by Astronomy Cast
- MESSENGER mission web site
- BepiColombo mission web site
Какой по счету Меркурий от Солнца?

Традиционно каждой планете присваивают свой номер, который говорит о том, как близко она находится к Солнцу. Каким же по счету среди планет Солнечной системы является Меркурий?
Меркурий ближе остальных планет к Солнцу, а потому он считается первой планетой. Расстояние от Меркурия до звезды изменяется от 46 до 69,7 млн км. При этом вторая планета от Солнца, которая носит имя Венеры, вращается на расстоянии 107-109 млн км от звезды.
Близость к Солнцу сказывается на облике Меркурия. Его поверхность может разогреваться до 427° С. На такой жаре плавятся многие металлы, например, цинк или олово. Также из-за перегрева Меркурий потерял почти всю свою атмосферу. Разогретые Солнцем молекулы газов с легкостью покидают планету и улетают в космос. Этот процесс называют диссипацией.
Чем ближе планета находится к своей звезде, тем большую скорость она развивает. Поэтому Меркурий оказывается быстрейшей планетой Солнечной системы. Даже свое имя он получил в честь древнеримского бога торговли, известного своей быстротой. Скорость движения Меркурия на орбите изменяется от 56,6 до 38,7 км/с, а ее среднее значение составляет 48 км/с. Один оборот вокруг звезды планета совершает всего лишь за 88 земных дней.
Близость к Солнцу мешает изучению Меркурия. Современные телескопы являются очень чувствительными приборами, поэтому многие из них просто нельзя направлять на Меркурий, так как мощный поток солнечного света испортит их. Также сложно вывести на меркурианскую орбиту спутник. Дело в том, гравитация самого Меркурия невелика (это самая легкая планета Солнечной системы) а вот гравитация Солнца около его орбиты наоборот, очень высока. Это мешает закрепиться исследовательским зондам на орбите планеты. Дополнительные сложности создает и высокая скорость передвижения Меркурия.
Список использованных источников
• https://cosmosplanet.ru/solnechnayasistema/merkurij/kakaya-po-schetu-planeta-merkurij-ot-solntsa.html
• https://spacegid.com/planetyi-nashey-s-vami-solnechnoy-sistemyi.html
Пришелец Инопланетянович
Если не оставишь коммент, то я приду за тобой!!!
Оставить коммент
Не нашли, то что искали? Используйте форму поиска по сайту
Понравилась статья? Оставь комментарий и поделись с друзьями
Солнечная система > Планета Меркурий
Исследование | Фотографии
- Введение
- Размер, масса и орбита
- Состав и поверхность
- Атмосфера и температура
- История изучения
- Исследование
Меркурий – первая планета от Солнца и самая маленькая планета в Солнечной системе. Это один из наиболее экстремальных миров. Свое название получил в честь посланника римских богов. Его можно отыскать без использования приборов, поэтому Меркурий отметился во многих культурах и мифах.
Однако это также и очень загадочный объект. Меркурий можно наблюдать утром и вечером в небе, а сама планета обладает собственными фазами.
Интересные факты
Давайте узнаем больше интересных фактов о планете Меркурий.
Год на Меркурии длится всего 88 дней
- Один солнечный день (промежуток между полуднями) охватывает 176 дней, а сидерический день (осевое вращение) – 59 дней. Меркурий наделен наибольшим орбитальным эксцентриситетом, а удаленность от Солнца – 46-70 млн. км.
Это наименьшая планета в системе
- Меркурия входит в пятерку планет, которые можно найти без использования инструментов. В экваторе простирается на 4879 км.
Стоит на втором месте по плотности
- Каждый см3 наделен показателем в 5.4 грамма. Но Земля стоит на первом месте, потому что Меркурий представлен тяжелыми металлами и горными породами.
Есть морщинки
- Когда железное планетарное ядро остыло и сжалось, поверхностный слой покрылся морщинками. Они способны вытягиваться на сотни миль.
Есть расплавленное ядро
- Исследователи считают, что железное ядро Меркурия способно пребывать в расплавленном состоянии. Обычно у маленьких планет оно быстро теряет нагрев. Но сейчас думают, что оно вмещает серу, которая снижает температуру плавления. Ядро охватывает 42% планетарного объема.
На втором месте по раскаленности
- Хотя Венера проживает дальше, но ее поверхность стабильно удерживает наивысшую поверхностную температуру из-за парникового эффекта. Дневная сторона Меркурия прогревается на 427°C, а на ночной температура падает к -173°C. Планета лишена атмосферного слоя, поэтому не способна обеспечивать равномерное распределение нагрева.
Наиболее кратерная планета
- Геологические процессы помогают планетам обновлять поверхностный слой и сглаживать кратерные шрамы. Но Меркурий лишен такой возможности. Все его кратеры именуются в честь художников, писателей и музыкантов. Ударные формирования, превышающие в диаметре 250 км, называют бассейнами. Крупнейший – Равнина Жары, простирающаяся на 1550 км.
Его посещали лишь два аппарата
- Меркурий слишком близко находится к Солнцу. Трижды его облетел Маринер-10 в 1974-1975 гг., отобразив чуть меньше половины поверхности. В 2004 году туда отправился MESSENGER.
Имя дали в честь посланника у римского божественного пантеона
- Точная дата обнаружения планеты неизвестна, потому что о ней писали еще шумеры в 3000 г. до н.э.
Есть атмосфера (кажется)
- Гравитация составляет лишь 38% от земной, но этого мало, чтобы удержать стабильную атмосферу (разрушается солнечными ветрами). Газ выходит, но его пополняют солнечные частички и пыль.
Хотите изменить жизнь к лучшему?
Опытный таролог ответит на вопросы:
Что ждёт Вас в будущем? Как сложатся отношения? Какое решение — верное?
Размер, масса и орбита
При радиусе в 2440 км и массе 3.3022 х 1023 кг Меркурий считается самой маленькой планетой в Солнечной системе. По размеру достигает всего 0.38 земного. Также уступает по параметрам некоторым спутникам, но по плотности стоит на втором месте после Земли – 5.427 г/см3. На нижнем фото указано сравнение размеров Меркурия и Земли.
Сравнение Меркурия и Земли
Это обладатель самой эксцентричной орбиты. Удаленность Меркурия от Солнца может колебаться от 46 миллионов км (перигелий) до 70 миллионов км (афелий). От этого могут меняться и ближайшие планеты. Средняя орбитальная скорость равна – 47322 км/с, поэтому на прохождения орбитального пути уходит 87.969 дней. Ниже представлена табличка характеристик планеты Меркурий.
Физические характеристики Меркурия
Экваториальный радиус | 2439,7 км |
---|---|
Полярный радиус | 2439,7 км |
Средний радиус | 2439,7 км |
Окружность большого круга | 15 329,1 км |
Площадь поверхности | 7,48·107 км² 0,147 земной |
Объём | 6,083·1010 км³ 0,056 земного |
Масса | 3,33·1023 кг 0,055 земной |
Средняя плотность | 5,427 г/см³ 0,984 земной |
Ускорение свободного падения на экваторе | 3,7 м/с² 0,377 g |
Первая космическая скорость | 3,1 км/с |
Вторая космическая скорость | 4,25 км/с |
Экваториальная скорость вращения | 10,892 км/ч |
Период вращения | 58,646 дней |
Наклон оси | 2,11′ ± 0,1′ |
Прямое восхождение северного полюса | 18 ч 44 мин 2 с 281,01° |
Склонение северного полюса | 61,45° |
Альбедо | 0,142 (Бонд) 0,068 (геом.) |
Видимая звёздная величина | от −2,6m до 5,7m |
Угловой диаметр | 4,5″ – 13″ |
Скорость оборота оси составляет 10.892 км/ч, поэтому сутки на Меркурии длятся 58.646 дней. Это говорит о том, что планета находится в резонансе 3:2 (3 осевых вращения на 2 орбитальных).
Эксцентричность и замедленность вращения приводят к тому, что планета тратит 176 дней на то, чтобы вернуться в изначальную точку. Так что один день на планете вдвое длиннее года. Также это обладатель наиболее низкого осевого наклона – 0.027 градусов.
Орбита и вращение Меркурия
Перигелий | 46 001 009 км 0,38709927 а. е. |
---|---|
Афелий | 69 817 445 км 0,46670079 а. е. |
Большая полуось | 57 909 227 км 0,38709927 а. е. |
Эксцентриситет орбиты | 0,20563593 |
Сидерический период обращения | 87,969 дней |
Синодический период обращения | 115,88 дней |
Орбитальная скорость | 47,36 км/с |
Средняя аномалия | 174,795884° |
Наклонение | 7,00° относительно плоскости эклиптики 3,38° относительно солнечного экватора 6,34° отн. инвариантной плоскости |
Долгота восходящего узла | 48,33167° |
Аргумент перицентра | 29,124279° |
Состав и поверхность
Состав Меркурия на 70% представлен металлическим и на 30% силикатным материалам. Считают, что его ядро охватывает примерно 42% всего объема планеты (у Земли – 17%). Внутри располагается ядро из расплавленного железа, вокруг которого сосредоточен силикатный слой (500-700 км). Поверхностный слой – кора с толщиной в 100-300 км. На поверхности можно заметить огромное количество хребтов, которые тянутся на километры.
По сравнению с другими планетами Солнечной системы, ядро Меркурия обладает наибольшим количеством железа. Полагают, что раньше Меркурий был намного больше. Но из-за удара с крупным объектом внешние слои разрушились, оставив главное тело.
Некоторые считают, что планета могла появиться в протопланетном диске до того, как солнечная энергия стала стабильной. Тогда он должен быть вдвое массивнее современного состояния. При нагреве в 25000-35000 К большая часть породы могла просто испариться. Изучите строение Меркурия на фото.
Внутренняя структура Меркурия представлена корой, мантией и ядром
Есть и еще одно предположение. Солнечная туманность могла привести к увеличению частичек, которые набросились на планету. Тогда более легкие отошли и не использовались при создании Меркурия.
Если смотреть издалека, то планета напоминает земной спутник. Такой же кратерный ландшафт с равнинами и следами лавовых потоков. Но здесь отмечено большее разнообразие элементов.
Хотите изменить жизнь к лучшему?
Опытный таролог ответит на вопросы:
Что ждёт Вас в будущем? Как сложатся отношения? Какое решение — верное?
Меркурий сформировался 4.6 миллиардов лет назад и попал под обстрел целой армии астероидов и мусорных осколков. Атмосферы не было, поэтому удары оставили заметные следы. Но планета оставалась активной, так что лавовые потоки создали равнины.
Улучшенные изображения кратеров Манч, Сандер и По среди вулканических равнин (оранжевые), недалеко от бассейна Калори
Размеры кратеров варьируются от небольших ям до бассейнов с шириною в сотни километров. Самый крупный – Калорис (равнина Жары) с диаметром в 1550 км. Удар был настолько сильным, что привел к лавовому извержению на противоположной планетарной стороне. А сам кратер окружен концентрическим кольцом высотой в 2 км. На поверхности можно отыскать примерно 15 крупных кратерных образований. Внимательно рассмотрите схему магнитного поля Меркурия.
Магнитное поле Меркурия
Планета обладает глобальным магнитным полем, достигающем 1.1% земной силы. Возможно, что источником служит динамо, напоминая нашу Землю. Оно образуется благодаря вращению жидкого ядра, наполненного железом.
Этого поля хватает, чтобы противостоять звездные ветра и формировать магнитосферный слой. Его силы достаточно, чтобы удерживать плазму из ветра, из-за чего происходит поверхностное выветривание.
Атмосфера и температура
Из-за близости к Солнцу планета слишком сильно прогревается, поэтому не способна сберечь атмосферу. Но ученые отметили тонкий слой переменной экзосферы, представленной водородом, кислородом, гелием, натрием, водяным паром и калием. Общий уровень давления приближается к отметке 10-14 бар.
Северный полюс планеты, запечатленный аппаратом MESSENGER. Красным цветом отмечены участки в тени, а желтый – лед
Без атмосферного слоя солнечное тепло не накапливается, поэтому на Меркурии отмечают серьезные температурные колебания: на солнечной стороне – 427°С, а на темной опускается до -173°С.
Однако поверхность располагает водяным льдом и органическими молекулами. Дело в том, что полюсные кратеры отличаются глубиной и туда не попадают прямые солнечные лучи. Полагают, что на дне можно обнаружить 1014 – 1015 кг льда. Пока нет точных данных о том, откуда на планете взялся лед, но это может быть подарок от упавших комет или же он происходит из-за дегазации воды от внутренней планетарной части.
История изучения планеты
Описание Меркурия не обходится без истории исследований. Эта планета доступна для наблюдения без использования приборов, поэтому фигурирует в мифах и древних легендах. Первые записи обнаружены в табличке Мул Апин, выступающей астрономическими и астрологическими вавилонскими записями.
Эти наблюдения сделаны в 14-м веке до н.э. и рассказывают о «пляшущей планете», потому что Меркурий перемещается быстрее всего. В Древней Греции его именовали Стилбон (переводится как «блеск»). Это был посланник Олимпа. Потом римляне переняли эту идею и дали современное наименование в честь своего пантеона.
Птолемей в работах несколько раз упоминал, что планеты способны проходить перед Солнцем. Но он не записывал в примеры Меркурий и Венеру, потому что считал их слишком маленькими и незаметными.
Китайцы именовали его Чэнь Синь («Часовая звезда») и связывали с водой и северной направленностью. Причем в азиатской культуре до сих пор сохранилось такое представление о планете, которую даже записывают как 5-й элемент.
Для германских племен здесь наблюдалась связь с богом Одином. Майя видели четырех сов, две из которых отвечали за утро, а две других за вечер.
О геоцентрическом орбитальном пути еще в 11 веке написал один из исламских астрономов. В 12-м веке Ибн Баджья отметил транзит двух крошечных темных тел перед Солнцем. Скорее всего он видел Венеру и Меркурий.
Проход Меркурия через солнечный диск, наблюдаемый SOHO в 2006 году. За транзитом можно было смотреть в Восточной Европе и восточном полушарии
Индийский астроном Кералы Сомаяджи в 15 веке создал частичную гелиоцентрическую модель, где Меркурий совершал обороты вокруг Солнца.
Первый обзор в телескоп приходится на 17 век. Это сделал Галилео Галилей. Он тогда внимательно изучал фазы Венеры. Но его аппарату не хватило мощности, поэтому Меркурий остался без внимания. А вот транзит отметил Пьер Гассенди в 1631 году.
Хотите изменить жизнь к лучшему?
Опытный таролог ответит на вопросы:
Что ждёт Вас в будущем? Как сложатся отношения? Какое решение — верное?
Орбитальные фазы в 1639 году заметил Джованни Зупи. Это было важное наблюдение, потому что подтвердило вращение вокруг звезды и правильность гелиоцентрической модели.
Более точные наблюдения в 1880-х гг. предоставил Джованни Скиапарелли. Он считал, что орбитальный путь занимает 88 дней. В 1934 году Юджиос Антониади создал детальную карту поверхности Меркурия.
Карта Меркурия, созданная Антониади
Первый радиолокационный сигнал удалось отбить советским ученым в 1962 году. Через три года американцы повторили эксперимент и закрепили осевой оборот в 59 дней. Обычные оптические наблюдения не смогли дать новых сведений, но интерферометры открыли химические и физические характеристики подповерхностных слоев.
Первое глубокое изучение поверхностных особенностей провели в 2000 году обсерваторией Маунт-Вильсон. Большую часть карты составили при помощи радиолокационного телескопа Аресибо, где расширение достигает 5 км.
Исследование планеты
До момента первого полета беспилотных аппаратов мы многого не знали о морфологических характеристиках. Первым к Меркурию отправился Маринер в 1974-1975 гг. Он трижды приблизился и сделал ряд масштабных фото.
Космический аппарат НАСА Маринер-10, который в 1970-х гг. исследовал Венеру и Меркурий
Но аппарат обладал длительным орбитальным периодом, поэтому при каждом приближении подходил к одной и той же стороне. Так что карта составляла лишь 45% всей площади.
При первом сближении удалось зафиксировать магнитное поле. Последующие подходы показали, что оно сильно напоминает земное, отклоняющее звездные ветры.
В 1975 году у аппарата кончилось топливо, и мы потеряли связь. Однако Маринер-10 и сейчас может вращаться вокруг Солнца и наведываться к Меркурию.
Вторым посланником стал MESSENGER. Он должен был разобраться в плотности, магнитном поле, геологии, структуре ядра и атмосферных особенностях. Для этого установили специальные камеры, гарантирующие высшее разрешение, а спектрометры отмечали составляющие элементы.
Аппарат MESSENGER вращается вокруг Меркурия с марта 2011 года
MESSENGER стартовал в 2004 году и выполнил три пролета с 2008 года, компенсировав упущенную Маринером-10 территорию. В 2011 году он перешел на эллиптическую планетарную орбиту и начал снимать поверхность.
После этого стартовала следующая годичная миссия. Последний маневр пришелся на 24 апреля 2015 года. После этого закончилось топливо, и 30 апреля спутник разбился об поверхность.
В 2016 году ЕКА и JAXA объединились для создания BepiColombo, который должен добраться к планете в 2024 году. У него есть два зонда, которые будут изучать магнитосферу, а также поверхность во всех длинах волн.
Расширенное изображение Меркурия, созданное на основе снимков камер MESSENGER
Меркурий – интересная планета, раздираемая крайностями и противоречиями. Обладает расплавленной поверхностью и льдом, нет атмосферы, зато присутствует магнитосфера. Мы надеемся, что будущие технологии позволят узнать больше интригующих подробностей. Обязательно рассмотрите, как выглядит современная карта поверхности Меркурия в высоком разрешении.
Карта поверхности
Нажмите на изображение, чтобы его увеличить
Полезные статьи:
- Интересные факты о Меркурии;
- Ближайшая к Солнцу планета;
- К какому типу планет принадлежит Меркурий?
- Ближайшая планета к Меркурию
- Возраст Меркурия
- Жизнь на Меркурии
- Обнаружение планеты Меркурий
- Кто открыл Меркурий?
- Посещали ли люди Меркурий?
- Как Меркурий получил свое имя?
- Терраформирование Меркурия
Положение и движение Меркурия
- Как далеко Меркурий от Солнца?
- Орбита Меркурия;
- Сколько лететь до Меркурия;
- Вращение Меркурия;
- Ретроградный Меркурий;
- Как долго длится день на Меркурии?;
- Год на Меркурии;
Ссылки
Меркурий — это самая маленькая планета Солнечной системы, расположенная максимально близко к небесному светилу. Однако он выделяется не только данной особенностью. Меркурий сложен для наблюдения с Земли, так как остается видимым для телескопов незадолго до наступления вечера или утра. Причем планета всегда располагается возле горизонта. Вследствие этого наблюдатель может рассмотреть только половину диска Меркурия.
Размер, масса и орбита Меркурия
Как было отмечено, Меркурий — самая маленькая планета Солнечной системы, общая масса которой достигает 3,3022х1023 кг. Его диаметр составляет 4879 км (радиус — 2440 км).
Интересный факт: диаметр Меркурия примерно в 3 раза меньше земного (12742 км), масса — в 18 раз меньше. По размерам данная планета уступает некоторым спутникам, которые встречаются в Солнечной системе.
Несмотря на указанные особенности, Меркурий считается плотной планетой. По этому показателю он только в 2 раза уступает Земле.
Меркурий отличается необычной орбитой. Максимально к Солнцу (перигелий) он приближается на расстояние в 46 млн км и удаляется на 70 млн (афелий). Из-за такой особенности Меркурий оказывает влияние на расположенные поблизости планеты, в частности, на Венеру.
Атмосфера
Большую часть Меркурия занимает плотное ядро, состоящее преимущественно из железа. Сверху присутствует сравнительно тонкий слой твердых пород, которые составляют поверхность. Атмосфера у этой планеты практически отсутствует: она в 1015 тоньше земной. Поэтому можно говорить, что над поверхностью Меркурия присутствует вакуум.
Причины отсутствия полноценной и ярко выраженной атмосферы точно не установлены. Существует предположение, что это обусловлено малой плотностью планеты, составляющей всего 30% от земной. Также это объясняется близким расположением к Солнцу, которое, испуская так называемый ветер, «сдувает» любые газы.
Проведенные исследования показывают, что тонкий слой над поверхностью планеты состоит из кислорода (42%), натрия (29%), водорода (22%), гелия (6%) и калия (0,5%). Также в атмосфере планеты в небольшом количестве содержатся магний, азот, ксенон, диоксид углерода, вода, неон, кальций и другие вещества.
Также определенную роль в том, что Меркурий практически лишен атмосферы, играют резкие перепады температур над поверхностью. Как показали исследования, этот показатель может достигать 610 градусов Цельсия (от -180 до +430).
Интересный факт: Меркурий является единственной планетой в Солнечной системе, у которой наблюдаются такие температурные колебания.
Определенную роль в этом играет как раз отсутствие многоуровневой атмосферы. На Земле плотные слои газов удерживают в течение ночи тепло. Но Меркурий из-за тонкой атмосферы не способен защищаться от солнечной радиации. В связи с этим, тепло практически сразу уходит в космическое пространство.
Температура
Отсутствие атмосферы делает Меркурий похожим на Луну. На первой планете, со стороны прогреваемой Солнцем, температура повышается до +430 градусов Цельсия. На темной области Меркурия поверхность быстро остывает. Ночью на планете температура опускается до -180 градусов.
Однако этим не ограничиваются особенности Меркурия. Поверхностный слой планеты рыхлый и отличается слабой теплопроводностью. Поэтому уже на глубине в метр температура породы стабилизируется на отметке в +75 градусов.
Вращение
По орбите Меркурий движения со средней скоростью 48 км/с. В связи с этим, год здесь длится порядка 88 дней. Однако скорость вращения вокруг своей оси составляет 10,892 км/ч. Вследствие этого сутки на Меркурии длятся чуть менее 59 земных дней.
Вращается первая планета не по стандартной орбите. Ученые установили, что Меркурий со временем постепенно смещается относительно Солнца. Данный эффект известен как прецессия.
Интересный факт: смещение орбиты Меркурия по отношению Солнца было одной из неразрешимых загадок, с которой столкнулись астрономы в 19-м веке. Чтобы объяснить данный эффект, ученые даже выдвинули предположение о том, что здесь располагается неизвестная планета, расположенная ближе к звезде.
Прецессия была объяснена ближе к 20-м годам XX века. К тому моменту Альберт Эйнштейн опубликовал Общую теорию относительности, которая позволяла понять, почему орбита Меркурия со временем смещается. Позднее данное предположение удалось подтвердить во время солнечного затмения.
Измерения показали, что спин-орбитальный резонанс Меркурия (количество оборотов, которое совершает планета на орбите) составляет 3:2. Из-за такой особенности возникает необычный эффект, который невозможно наблюдать на Земле. Если бы человек находился на поверхности Меркурия, то смог бы увидеть, как Солнце поднимается до максимальной точки на небосводе, затем по той же траектории опускается к горизонту.
День и ночь
Поскольку Меркурий медленно вращается вокруг собственной оси, одно из полушарий планеты в течение продолжительного срока обращено в сторону Солнца. В связи с этим, смена дня и ночи происходит крайне редко. У Меркурия данный процесс занимает существенно больше времени, чем на других планетах Солнечной системы.
Интересный факт: день или ночь здесь длятся ровно столько же, сколько и год. Объясняется это тем, что длина суток не намного меньше продолжительности движения планеты по орбите (88 дней).
Ось Меркурия отклонена от орбиты всего на 7 градусов. Данная особенность приводит к тому, что на планете не наблюдается смены времен года. По той же причине в районе полюсов есть зоны, на которые никогда не попадает солнечный свет.
Поверхность планеты Меркурий
Поверхность Меркурия
Поверхность Меркурия
Поверхность Меркурия
Поверхность Меркурия
Поверхность Меркурия
До 1974 года поверхность Меркурия оставалась загадкой. Во многом это объяснялось сложностями наблюдения за планетой с Земли. Но благодаря фотографиям, полученным в 1974 году после трехкратного облета космического аппарата «Маринер-10», особенности рельефа Меркурия были раскрыты.
Меркурий отличается сложным рельефом, образованным многочисленными кратерами, которые получились из-за частых столкновений с метеоритами в прошлом. При этом поверхность между ними оказалась очень ровной. Данная особенность обусловлена движением потоков лавы, которая извергалась на планете в далеком прошлом. Кроме того, снимки показали, что поверхность Меркурия усеяна множеством скал, протяженность которых достигает нескольких тысяч километров, высота — не более двух.
Интересный факт: наличие протяженных ровных участков свидетельствует о том, что планета в прошлом была геологически активна. Но такое количество кратеров указывает на обратное. Ученые пока не смогли объяснить это противоречие.
Еще одна особенность заключается в том, что скалы на Меркурии образуются не так, как на Земле. На последней они появляются из-за смещения тектонических плит. Но поверхность Меркурия вспучивается из-за постепенного сжатия ядра. Как показали результаты исследований, за время существования планеты ее диаметр из-за указанного процесса уменьшился примерно на 1,5 км.
Магнитное поле
В рамках исследования, проведенного в 1974 году, было установлено, что напряженность магнитного поля Меркурия составляет примерно 300 нТл. Этот показатель в 100 раз меньше земного.
Магнитное поле первой планеты характеризуется дипольной структурой и высокой симметричностью. Оно незначительно (всего на 10 градусов) отклоняется от оси вращения, что усложняет определение природы его появления. Считается, что образованию магнитного поля Меркурия способствует эффект динамо. То есть оно образуется благодаря тому же процессу, который наблюдается на Земле.
Считается, что магнитное поле сформировано из-за циркуляции веществ в жидком ядре планеты. Причем из-за близкого расположения Меркурия к Солнцу возникает очень сильный приливной эффект. Благодаря этому ядро планеты остается жидким, что необходимо для образования магнитного поля. Согласно проведенным расчетам, последнее появилось примерно 3,7-3,9 млрд лет назад.
Несмотря на сравнительно малую напряженность, магнитное поле Меркурия способно оказывать влияние на движение солнечного ветра. Из-за этого возникает так называемая магнитосфера, которая также отличается небольшими размерами.
В 2003 году стало известно, что из-за близкого расположения к Солнцу его ветер образует над поверхностью Меркурия необычные эффекты. Во-первых, в магнитосфере выявлены так называемые «окна», или зоны, появление которых обусловлено снижением напряженности поля. Во-вторых, солнечный ветер вызывает образование завихрений. Последние представляют собой сплетенные узлы магнитного поля планеты, которые вытягиваются на расстояние в 800 км.
Кратеры
Исследования Меркурия показали, что поверхность усеяна кратерами разных размеров. Рельеф планеты составляют как незначительные впадины, напоминающие чаши, так и крупные формирования, достигающие нескольких сотен километров в поперечнике. Часть кратеров успели к настоящему моменту сильно разрушиться. Но есть формирования, которые сохранили первоначальную структуру.
Интересный факт: кратеры на Меркурии обладают меньшим размером, чем у Луны. Такая разница обусловлена более высокой силой притяжения на планете.
Среди примечательных деталей рельефа Меркурия часто упоминают равнину Жары, образовавшуюся вследствие вулканической активности. Стекавшая в прошлом лава заполнила крупнейший кратер на планете размером 1525 х 1315 км. В его центре пролегает система борозд, названная Пантеон. Периметр кратера достигает высоты более двух километров.
Согласно теории, описывающей его образование, он появился из-за столкновения с небесным телом диаметром в 100 км. Сила удара была настолько велика, что возникшие в этот момент сейсмические волны прошли через всю планету.
Другой кратер, названный Койпером, является самой яркой зоной на Меркурии. Длина этого участка достигает 60 км.
Структура
Верхний слой Меркурия – это кора, толщина которой составляет от 100 до 300 км. Присутствие на поверхности небесного тела выпуклостей указывает на ее сходство с Землей. То есть кора Меркурия достаточно хрупкая.
Средний слой планеты составляет мантия, толщина которой достигает 600 км. В сравнении с другими небесными телами, у Меркурия она относительно тонкая. Ученые объясняют этот факт тем, что планета в раннюю историю своего существования столкнулась с так называемым планетезималием, из-за чего существенно уменьшилась масса мантии.
В центре Меркурия располагается ядро диаметром 3,6 тысяч км. Оно обладает несколькими необычными свойствами, наиболее примечательным из которых является плотность. При диаметре планеты в 4878 км данный показатель равен 5540 кг/м3.
Столь высокая плотность Меркурия объясняется только одной причиной: его ядро жидкое. Об этом свидетельствуют и результаты проведенного исследования, в рамках которого оценивался характер отскока радиоволн от поверхности планеты.
Возраст Меркурия
Точный возраст Меркурия неизвестен. Но он, вероятно, образовался примерно в тот же период, что и остальные планеты солнечной системы. На это указывают результаты радиоуглеродного анализа. На основе данных ученые установили, что Меркурий появился около 4,6 млрд лет назад.
Жизнь на Меркурии
Для зарождения жизни на Меркурии неподходящие условия. Чтобы на планете появились организмы, способные воспроизводить себе подобных, необходимо соблюдение ряда условий. В частности нужно, чтобы вода в течение длительного времени не замерзала и не испарялась. Но на Меркурии наблюдаются значительные колебания температур, из-за которых невозможно зарождение жизни. Кроме того, этому помешала масштабная бомбардировка планеты метеоритами, которая произошла примерно 3,9 млрд лет назад.
Обнаружение планеты
Точная дата обнаружения Меркурия неизвестна. Ученые нашли упоминание об этой планете в шумерской письменности, которая относится ко второму тысячелетию до нашей эры. Позднее Меркурий отмечали в трудах древних греков и римлян, ученые из Средневековья и эпохи Возрождения.
Вода на Меркурии
Несмотря на внушительные температурные колебания, вода на Меркурии есть. Она представлена в виде льда, который находится в кратерах, расположенных на полюсах планеты. Вода здесь не испаряется, так как сюда не проникают солнечные лучи.
Как далеко Меркурий от Солнца?
Как говорилось выше, расстояние Меркурия от Солнца — это непостоянная величина. Данный показатель зависит от места нахождения планеты на орбите. Меркурий может приближаться к Солнцу на расстояние чуть более 46 млн км и удаляться на 70 млн км.
Сколько лететь до Меркурия?
Продолжительность полета от Земли до Меркурия зависит от характеристик аппарата. Свет преодолевает это расстояние за 4,3 минуты. Отправленный в 1974 году летательный аппарат «Маринер-10» достиг Меркурия за 147 дней. Спустя 40 лет орбиты планеты достиг другой искусственный спутник – «Мессенджер». Ему на это потребовалось 1260 дней.
Почему Меркурий так называется?
Названия многих небесных тел происходят из древнеримского божественного пантеона. Причина, почему именно Меркурий получил такое имя, неизвестна. Более того, в зависимости от источника планета называлась по-разному. В письменности древних греков ее упоминали как Гермес и Аполлон. В Вавилоне эта планета была известна в качестве Напу. Но официальное название закрепилось после римлян, которые отождествляли Меркурий с богом торговли.
Характеристики
Меркурий отличается следующими характеристиками:
- плотность — 5430 кг/м3;
- масса — 0,055 земного веса (3,3*1023 кг);
- диаметр экватора — 4880 км;
- наклон оси — 0,01 градуса;
- длина суток — 59 земных дней;
- средняя температура на поверхности планеты – -73 градуса;
- среднее расстояние до солнца — 58 млн км;
- продолжительность года — 88 земных дней;
- скорость вращения вдоль орбиты — 48 км/с;
- эксцентриситет орбиты — 0,0206;
- наклон орбиты к эклиптике — 7 градусов;
- скорость свободного падения — 3,7 м/с2.
У Меркурия отсутствуют спутники.
История изучения и исследований
Близость расположения к небесному светилу мешает изучению Меркурия. Первые четкие фотографии планеты были получены в 1974 году, когда космический зонд «Маринер-10» осуществил 3 пролета над ней. Спустя более чем 40 лет до Меркурия добрался аппарат «Мессенджер». Он тоже совершил облет планеты, и спустя 7 лет он закрепился на орбите планеты. Благодаря данному зонду ученые получили информацию о магнитном поле, атмосфере и особенностях рельефа планеты.
Интересное видео о Меркурии
Если Вы нашли ошибку, пожалуйста, выделите фрагмент текста и нажмите Ctrl+Enter.
Планеты Солнечной системы называются так потому, что они располагаются рядом с Солнцем и вращаются вокруг этой звезды.
В список этих планет входят:
- Меркурий,
- Венера,
- Земля,
- Марс,
- Юпитер,
- Сатурн,
- Уран,
- Нептун.
Именно в таком порядке они расположены от Солнца. Меркурий — ближайшая к Солнцу планета, Нептун — самая дальняя. Как видно, Земля — третья по счёту планета от Солнца.
Все планеты обращаются вокруг Солнца со своей скоростью. Скорость обращения Земли составляет 107 км/ч. У Меркурия — 170 км/ч, а у Нептуна — 19 км/ч. Выходит, что чем ближе планета находится к Солнцу, тем выше скорость её обращения по орбите.
К тому же почти все планеты (кроме Меркурия) имеют атмосферу. То есть оболочку, которая состоит из газа и окружает планету.
Что стало с Плутоном?
До 2006 года планет в Солнечной системе было девять. Но после того как Международный астрономический союз внёс коррективы в понятие «планета», Плутон перестал быть планетой и стал «карликовой» планетой.
Так, союз установил три критерия, чтобы небесное тело могло называться планетой:
- Орбита планеты проходит вокруг Солнца, т. е. планета обращается только вокруг этой звезды.
- Небесное тело должно быть достаточно крупным, гравитация этого тела должна придавать ему форму шара.
- Гравитационное поле планеты должно быть настолько сильным, чтобы превратить все небесные тела на своей орбите в свои спутники или вытолкнуть их за пределы орбиты.
Получается, что Плутон недостаточно большой и к тому же имеет другие небесные тела вокруг своей орбиты.
Каковы размеры планет?
Название | Экваториальная окружность | Порядковый номер по размеру |
---|---|---|
Меркурий | 15 км | № 8 |
Венера | 38 км | № 6 |
Земля | 40 км | № 5 |
Марс | 21 км | № 7 |
Юпитер | 439 км | № 1 |
Сатурн | 365 км | № 2 |
Уран | 159 км | № 3 |
Нептун | 154 км | № 4 |
Как видно, Меркурий — самая маленькая планета, а Юпитер — самая большая.
Узнайте больше про Солнечную систему.
Чтобы лучше понять размеры каждой планеты, смотрите видео телестудии Роскосмоса. В нём показывают, как смотрелось бы небо, если спутником Земли была не Луна, а другие планеты.
Как выглядят планеты Солнечной системы?
Меркурий
Самая ближайшая к Солнцу планета, расстояние между ними составляет 58 млн км. Планета названа в честь древнеримского бога торговли Меркурия. Спутников у неё нет.
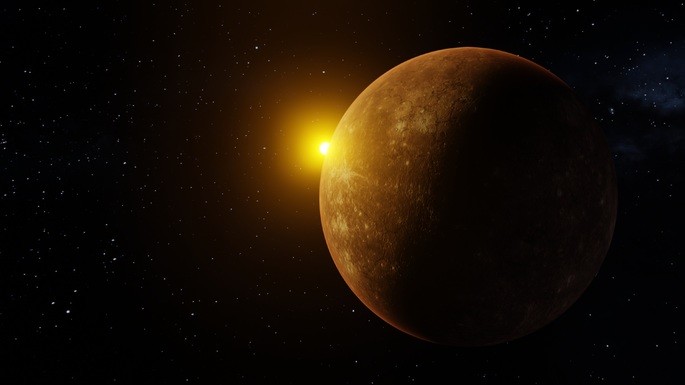
Меркурий вращается вокруг своей оси медленно, а вокруг Солнца — быстро (170 км/ч). Чтобы увидеть один восход и один закат (астрономические сутки) Меркурию нужно сделать два оборота вокруг Солнца.
Чтобы обернуться вокруг своей оси (сидерический день) Меркурию нужно 59 земных суток. А астрономические сутки длятся 176 земных суток. Полный оборот вокруг Солнца планета делает за 88 земных суток.
По предположительным подсчетам на этой планете в течение дня температура бывает больше 400 градусов Цельсия выше нуля, а ночью — почти минус 200.
Масса Меркурия — 0,055 массы Земли. Если провести эксперимент и отправить человека весом 80 кг на Меркурий, то там он будет весить всего 30 кг. Это объясняется тем, что у планет разная масса, сила притяжения и размер.
Меркурий своей поверхностью напоминает Луну. Она скалистая, покрыта кратерами. Экзосфера планеты состоит из кислорода, натрия, водорода, гелия и калия.
Венера
Вторая планета от Солнца, находится на расстоянии 108 млн км. Своё название она получила в честь древнеримской богини любви, красоты и плодородия Венеры. Спутников нет.
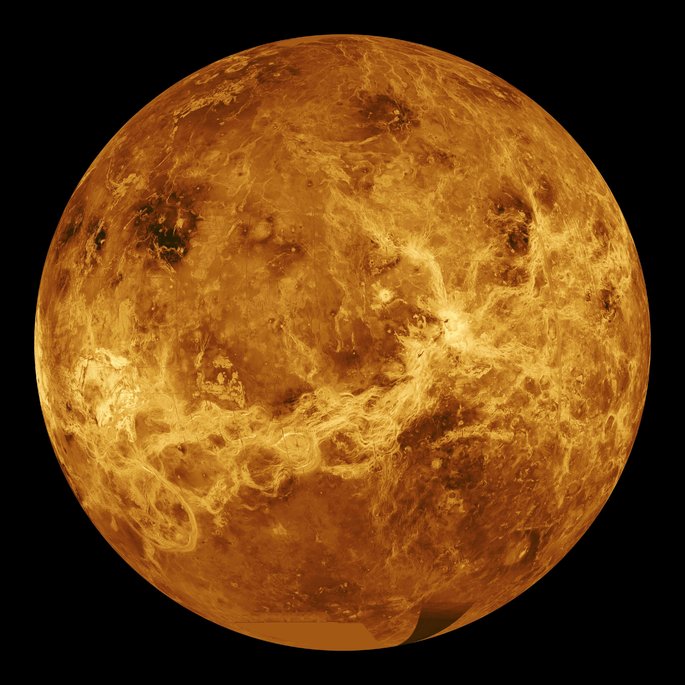
Скорость вращения по орбите — 126 км/ч. Один сидерический день на планете — 243 земных суток. Но встаёт Солнце на западе, а заходит на Востоке, потому что Венера вращается в обратную сторону. Один год на Венере — это почти 225 земных суток.
Температура поверхности — 465 градусов Цельсия выше нуля.
Масса Венеры — 0,815 массы Земли. По размеру Венера почти как Земля. Человек, который весит 80 кг, на Венере будет весить 72,5 кг.
На Венере есть вулканы и кратеры. Атмосфера состоит в основном из углекислого газа (97%) и азота (3%).
Узнайте больше про Венеру.
Земля
Третья планета от Солнца или голубая планета. Удалена от звезды на 150 млн км. Название пришло от англо-саксонского слова erda (в современном английском языке — Earth, что значит «грунт», «земля»). Есть спутник — Луна.
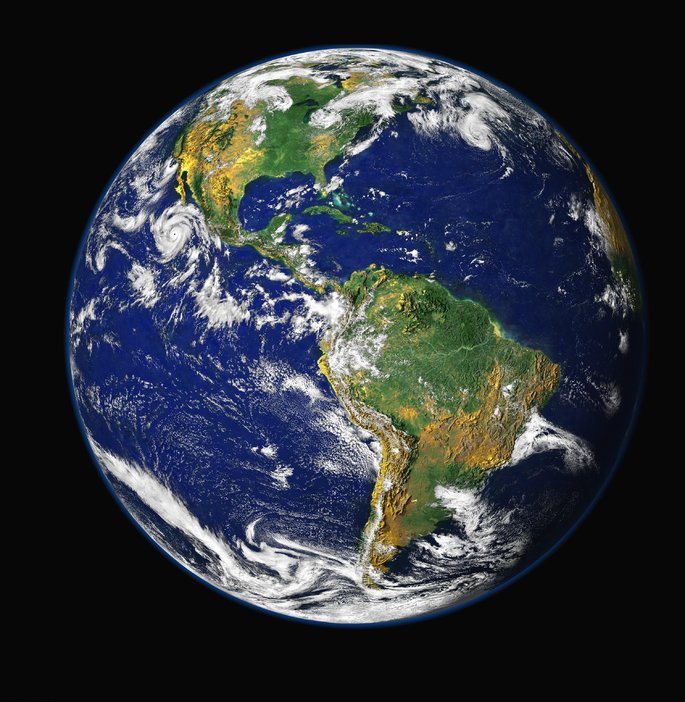
Скорость обращения вокруг Солнца — 107 км/ч. Один оборот вокруг звезды наша планета делает за 23 часа и 56 минут.
Атмосфера Земли наполнена азотом (78,1%) и кислородом (21%).
Узнайте больше про Землю.
Марс
Четвёртая планета. До Солнца — 228 млн км, названа в честь древнеримского бога войны. У Марса два спутника — Деймос и Фобос.
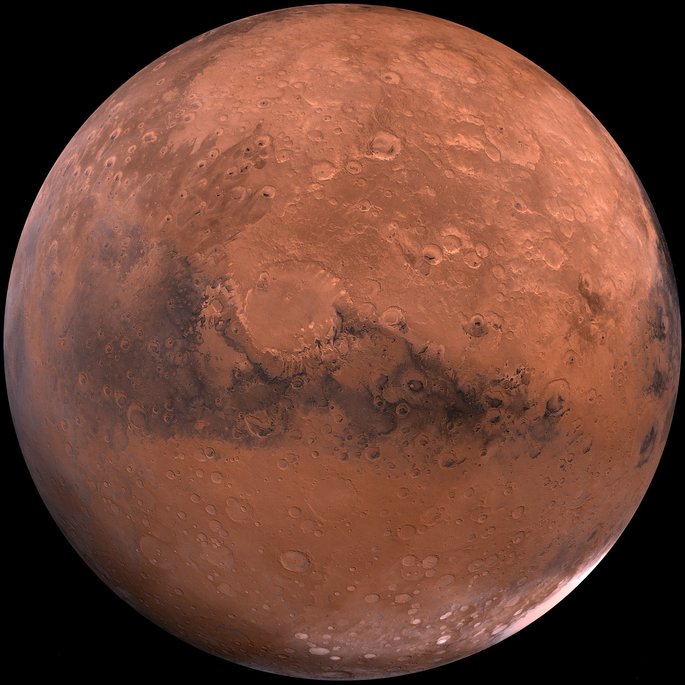
Скорость обращения вокруг Солнца — 86,6 км/ч. Оборот вокруг своей оси Марс делает примерно за то же время, что и Земля — 24,6 часов. А вот полный оборот вокруг Солнца — за 687 дней.
Средняя температура на этой планете минус 27 градусов Цельсия. Но на Марсе есть времена года, один сезон сменяет другой.
Марс в два раза меньше Земли. 80-килограммовый человек будет весить на Марсе 30 кг.
Её ещё называют красной планетой, из-за оксида железа (маггемита), который покрывает поверхность. В атмосфере 95% занимает углекислый газ, азот (2,5%) и аргон (около 2%). Присутствует и вода, но в очень маленьком количестве (до 0,1%).
Узнайте больше про Марс.
Юпитер
Пятая и самая большая планета. Расстояние до Солнца — 778 млн км. Названа в честь древнеримского бога-громовержца. У Юпитера 79 спутников, есть и кольцо, которое опоясывает планету. Но оно очень слабо различимое. Обнаружить его удалось только с помощью зонда, который подлетел близко к планете.
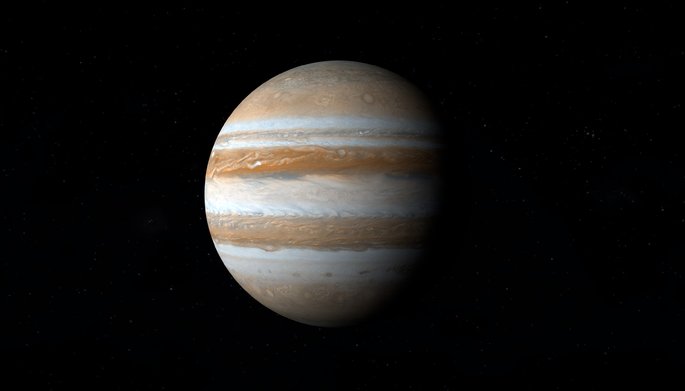
Скорость обращения — 47 км/ч; ему нужно 12 земных лет, чтобы сделать полный круг вокруг Солнца.
Масса Юпитера в 318 раз больше массы Земли. 80-килограммовый человек будет весить 189 кг на этой планете.
Юпитер называют газовым гигантом, потому что он состоит в основном из водорода и гелия, а поверхность у него нетвёрдая. Вокруг планеты бушуют бури, самая мощная из них — Большое красное пятно. Эта буря не успокаивается уже более 300 лет.
Узнайте больше про Юпитер.
Сатурн
Шестая планета, которая находится на расстоянии 1,4 млрд км от Солнца. Она названа в честь древнеримского бога земледелия.
У Сатурна семь больших колец, они состоят изо льда и камней. Их ширина — 400 000 км.
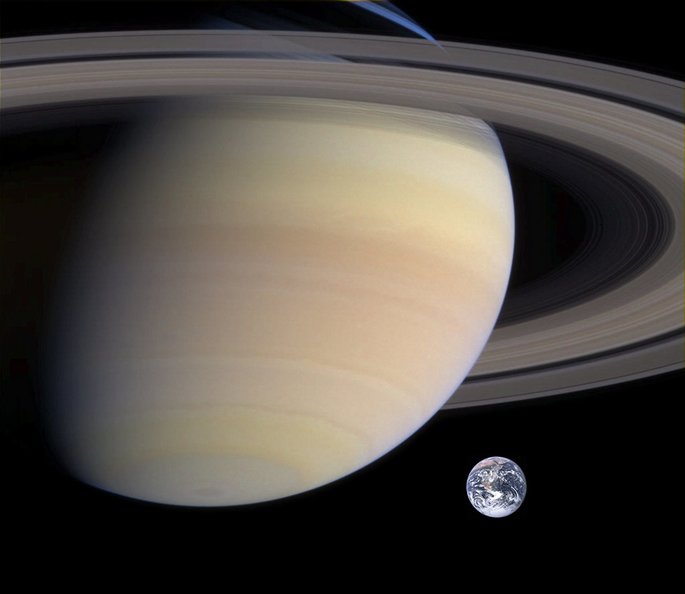
По данным НАСА, у планеты 82 признанных спутника.
Скорость обращения — 34 км/ч. Год на Сатурне — это 29 земных лет. А сидерический день — примерно 10 часов.
По массе она в 95 раз больше Земли. Продолжая наш эксперимент с килограммами, вес 80-килограммового человека на Сатурне снизится до 73 кг.
Сатурн похож на Юпитер своим составом. Это тоже газовый гигант, его атмосфера состоит из водорода и гелия.
Узнайте больше про Сатурн.
Уран
Седьмая планета, расположенная в 2,8 млрд км от Солнца. Названа в честь древнегреческого бога неба. Эта первая планета, которую открыли после того, как был изобретён телескоп.
У Урана 27 спутников и тоже есть кольца.
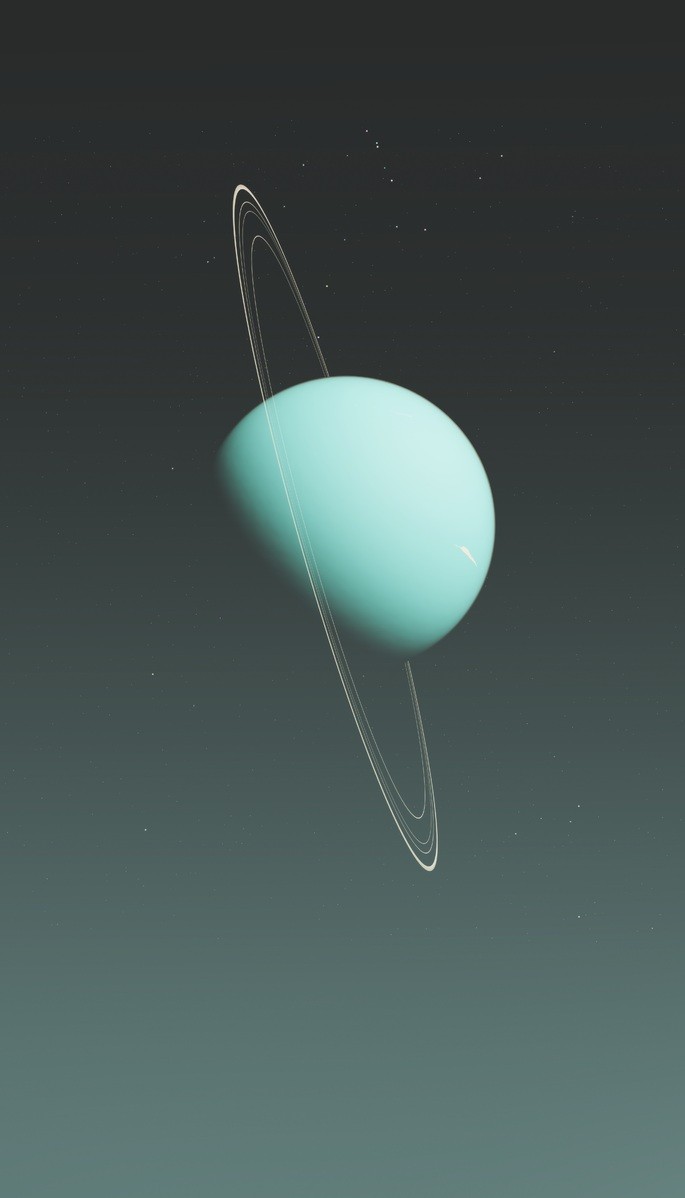
Скорость обращения — 24 км/ч. Ему нужно 84 земных лет, чтобы пройти весь путь по своей орбите вокруг Солнца. День на Уране — 17 часов на Земле.
Планета в 14,5 раз больше Земли по массе. 80-килограммовый человек будет весить 71 кг на Уране.
Уран — ледяной гигант. Атмосфера состоит из молекул водорода, атомов гелия и небольшого количества метана.
Нептун
Ещё один ледяной гигант и восьмая планета. Удалена от Солнца на 4,5 млрд км. Названа в честь древнеримского бога морей.
У планеты 14 спутников, есть и кольца.
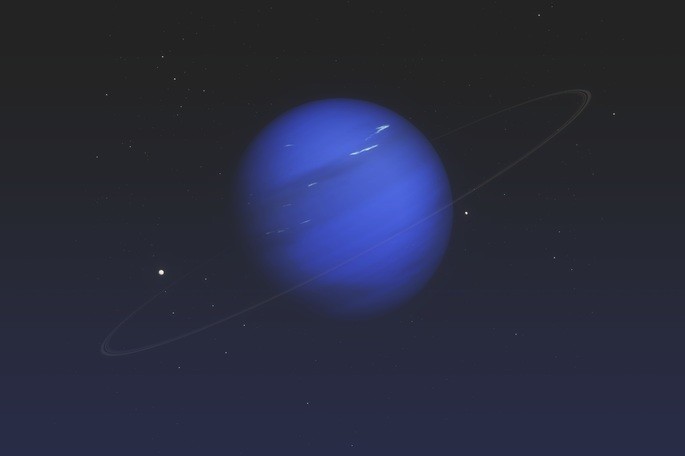
Скорость обращения — 19,5 км/ч. Нептуну понадобится 165 земных лет для того, чтобы совершить оборот вокруг Солнца.
Масса в 17,2 раз больше массы Земли. 80-килограммовый человек будет весить 90 кг на Нептуне.
Как и в случае с Ураном, атмосфера планеты состоит из молекул водорода, атомов гелия и метана.
Узнайте больше про Солнце, также, что такое Галактика и из чего состоит Атмосфера Земли.
Планеты Солнечной системы
Согласно официальной позиции Международного астрономического союза (МАС), организации присваивающей имена астрономическим объектам, планет всего 8.
Содержание:
- 1 Текущее положение среди планет Солнечной системы
- 2 Планеты Солнечной системы по порядку
- 3 Планеты земного типа
- 3.1 Меркурий
- 3.2 Венера
- 3.3 Земля
- 3.4 Марс
- 4 Наглядная модель Солнечной системы
- 5 Солнце
- 6 Меркурий
- 7 Венера
- 8 Земля
- 9 Марс
- 10 Юпитер
- 11 Сатурн
- 12 Уран
- 13 Нептун
- 14 Плутон
- 15 Планеты — гиганты
- 15.1 Юпитер
- 15.2 Сатурн
- 15.3 Уран
- 15.4 Нептун
Текущее положение среди планет Солнечной системы
Плутон был исключен из разряда планет в 2006 году. т.к. в поясе Койпера находятся объекты которые больше/либо равны по размерам с Плутоном. Поэтому, даже если его принимать его за полноценное небесное тело, то тогда необходимо к этой категории присоединить Эриду, у которой с Плутоном почти одинаковый размер.
Планеты Солнечной системы по порядку
По определению MAC, есть 8 известных планет: Меркурий, Венера, Земля, Марс, Юпитер, Сатурн, Уран и Нептун.
Все планеты делят на две категории в зависимости от их физических характеристик: земной группы и газовые гиганты.
Схематическое изображение расположения планет
Планеты земного типа
Меркурий
Самая маленькая планета Солнечной системы имеет радиус всего 2440 км. Период обращения вокруг Солнца, для простоты понимания приравненный к земному году, составляет 88 дней, при этом оборот вокруг собственной оси Меркурий успевает совершить всего полтора раза. Таким образом, его сутки длятся приблизительно 59 земных дней. Долгое время считалось, что эта планета все время повёрнута к Солнцу одной и той же стороной, поскольку периоды его видимости с Земли повторялись с периодичностью, примерно равной четырем Меркурианским суткам. Это заблуждение было развеяно с появлением возможности применять радиолокационные исследования и вести постоянные наблюдения с помощью космических станций. Орбита Меркурия – одна из самых нестабильных, меняется не только скорость перемещения и его удалённость от Солнца, но и само положение. Любой интересующийся может наблюдать этот эффект.
Меркурий в цвете, снимок космического аппарата MESSENGER
Близость к Солнцу стала причиной того, что Меркурий подвержен самым большим перепадам температуры среди планет нашей системы. Средняя дневная температура составляет около 350 градусов по Цельсию, а ночная -170 °C. В атмосфере выявлены натрий, кислород, гелий, калий, водород и аргон. Существует теория, что он был ранее спутником Венеры, но пока это остается недоказанным. Собственные спутники у него отсутствуют.
Венера
Вторая от Солнца планета, атмосфера которой почти полностью состоит из углекислого газа. Её часто называют Утренней звездой и Вечерней звездой, потому что она первой из звёзд становится видна после заката, так же как и перед рассветом продолжает быть видимой и тогда, когда все остальные звёзды скрылись из поля зрения. Процент диоксида углерода составляет в атмосфере 96%, азота в ней сравнительно немного – почти 4% и в совсем незначительном количестве присутствует водяной пар и кислород.
Венера в УФ спектре
Подобная атмосфера создает эффект парника, температура на поверхности из-за этого даже выше, чем у Меркурия и достигает 475 °C. Считается самой неторопливой, венерианские сутки длятся 243 земных дня, что почти равно году на Венере – 225 земных дней. Многие называют её сестрой Земли из-за массы и радиуса, значения которых очень близки к земным показателям. Радиус Венеры составляет 6052 км (0,85% земного). Спутников, как и у Меркурия, нет.
Земля
Третья планета от Солнца и единственная в нашей системе, где на поверхности есть жидкая вода, без которой не смогла бы развиться жизнь на планете. По крайней мере, жизнь в том виде, в котором мы её знаем. Радиус Земли равен 6371 км и, в отличие от остальных небесных тел нашей системы, более 70% её поверхности покрыто водой. Остальное пространство занимают материки. Ещё одной особенностью Земли являются тектонические плиты, скрытые под мантией планеты. При этом они способны перемещаться, хоть и с очень малой скоростью, что со временем вызывает изменение ландшафта. Скорость перемещения планеты по ней – 29-30 км/сек.
Наша планета из космоса
Один оборот вокруг своей оси занимает почти 24 часа, причем полное прохождение по орбите длится 365 суток, что намного больше в сравнении с ближайшими планетами-соседями. Земные сутки и год также приняты как эталон, но сделано это лишь для удобства восприятия временных отрезков на остальных планетах. У Земли имеется один естественный спутник – Луна.
Марс
Марс, снимок космического телескопа Хаббл в 2003 году
Четвёртая планета от Солнца, известная своей разрежённой атмосферой. Начиная с 1960 года, Марс активно исследуется учеными нескольких стран, включая СССР и США. Не все программы исследования были успешными, но найденная на некоторых участках вода позволяет предположить, что примитивная жизнь на Марсе существует, или существовала в прошлом.
Яркость этой планеты позволяет видеть его с Земли без всяких приборов. Причем раз в 15-17 лет, во время Противостояния, он становится самым ярким объектом на небе, затмевая собой даже Юпитер и Венеру.
Радиус почти вдвое меньше земного и составляет 3390 км, зато год значительно дольше – 687 суток. Спутников у него 2 — Фобос и Деймос.
Наглядная модель Солнечной системы
Внимание! Анимация работает только в браузерах поддерживающих стандарт -webkit (Google Chrome, Opera или Safari).
- Солнце
- Меркурий
- Венера
- Земля & Moon
- Марс
- Астероиды & Метеориты
- Юпитер
- Saturn & Ring
- Уран
- Нептун
- Плутон
-
Солнце
Солнце является звездой, которая представляет собой горячий шар из раскаленных газов в центре нашей Солнечной системы. Его влияние простирается далеко за пределы орбит Нептуна и Плутона. Без Солнца и его интенсивной энергии и тепла, не было бы жизни на Земле. Существуют миллиарды звезд, как наше Солнце, разбросанных по галактике Млечный Путь.
-
Меркурий
Выжженный Солнцем Меркурий лишь немного больше, чем спутник Земли Луна. Подобно Луне, Меркурий практически лишен атмосферы и не может сгладить следы воздействия от падения метеоритов, поэтому он как и Луна покрыт кратерами. Дневная сторона Меркурия очень сильно нагревается на Солнце, а на ночной стороне температура падает на сотни градусов ниже нуля. В кратерах Меркурия, которые расположены на полюсах, существует лед. Меркурий совершает один оборот вокруг Солнца за 88 дней.
-
Венера
Венера это мир чудовищной жары (еще больше чем на Меркурии) и вулканической активности. Аналогичная по структуре и размеру Земле, Венера покрыта толстой и токсичной атмосферой, которая создает сильный парниковый эффект. Этот выжженной мир достаточно горячий, чтобы расплавить свинец. Радарные снимки сквозь могучую атмосферу выявили вулканы и деформированные горы. Венера вращается в противоположном направлении, от вращения большинства планет.
-
Земля
Земля — планета океан. Наш дом, с его обилием воды и жизни делает его уникальным, в нашей Солнечной системе Земля занимает особое место. Другие планеты, в том числе несколько лун, также имеют залежи льда, атмосферу, времена года и даже погоду, но только на Земле все эти компоненты собрались вместе таким образом, что стало возможным существование жизнь.
-
Марс
Хотя детали поверхности Марса трудно увидеть с Земли, наблюдения в телескоп показывают, что на Марсе существуют сезоны и белые пятна на полюсах. В течение многих десятилетий, люди полагали, что яркие и темные области на Марсе это пятна растительности и что Марс может быть подходящим местом для жизни, и что вода существует в полярных шапках. Когда космический аппарат Маринер-4, прилетел у Марсу в 1965 году, многие из ученых были потрясены, увидев фотографии мрачной планеты покрытой кратерами. Марс оказался мертвой планетой. Более поздние миссии, однако, показали, что Марс хранит множество тайн, которые еще предстоит решить.
-
Юпитер
Юпитер — самая массивная планета в нашей Солнечной системе, имеет четыре больших спутника и множество небольших лун. Юпитер образует своего рода миниатюрную Солнечную систему. Чтобы превратится в полноценную звезду, Юпитеру нужно было стать в 80 раз массивнее.
-
Сатурн
Сатурн — самая дальняя из пяти планет, которые были известны до изобретения телескопа. Подобно Юпитеру, Сатурн состоит в основном из водорода и гелия. Его объем в 755 раз больше, чем у Земли. Ветры в его атмосфере достигают скорости 500 метров в секунду. Эти быстрые ветра в сочетании с теплом, поднимающимся из недр планеты, вызывают появление желтых и золотистых полос, которые мы видим в атмосфере.
-
Уран
Первая планета найденная с помощью телескопа, Уран был открыт в 1781 году астрономом Уильямом Гершелем. Седьмая планета от Солнца настолько далека, что один оборот вокруг Солнца занимает 84 года.
-
Нептун
Почти в 4,5 млрд. километрах от Солнца вращается далекий Нептун. На один оборот вокруг Солнца у него уходит 165 лет. Он невидим невооруженным глазом из-за его огромного расстояния от Земли. Интересно, что его необычная эллиптическая орбита, пересекается с орбитой карликовой планеты Плутона из-за чего Плутон находится внутри орбиты Нептуна порядка 20 лет из 248 за которые совершает один оборот вокруг Солнца.
-
Плутон
Крошечный, холодный и невероятно далекий Плутон был открыт в 1930 году и долго считался девятой планетой. Но после открытий подобных Плутону миров, которые находились еще дальше, Плутон был переведен в категорию карликовых планет в 2006 году.
Планеты — гиганты
Существуют четыре газовых гиганта, располагающихся за орбитой Марса: Юпитер, Сатурн, Уран, Нептун. Они находятся во внешней Солнечной системе. Отличаются своей массивностью и газовым составом.
Планеты солнечной системы, масштаб не соблюден
Юпитер
Пятая по счёту от Солнца и крупнейшая планета нашей системы. Радиус её – 69912 км, она в 19 раз больше Земли и всего в 10 раз меньше Солнца. Год на Юпитере не самый долгий в солнечной системе, длится 4333 земных суток (неполных 12 лет). Его же собственные сутки имеют продолжительность около 10 земных часов. Точный состав поверхности планеты пока определить не удалось, однако известно, что криптон, аргон и ксенон имеются на Юпитере в гораздо больших количествах, чем на Солнце.
Юпитер, снимок зонда Вояджер-1
Существует мнение, что один из четырёх газовых гигантов на самом деле – несостоявшаяся звезда. В пользу этой теории говорит и самое большое количество спутников, которых у Юпитера много – целых 67. Чтобы представить себе их поведение на орбите планеты, нужна достаточно точная и чёткая модель солнечной системы. Самые крупные из них – Каллисто, Ганимед, Ио и Европа. При этом Ганимед является крупнейшим спутником планет во всей солнечной системе, радиус его составляет 2634 км, что на 8% превышает размер Меркурия, самой маленькой планеты нашей системы. Ио отличается тем, что является одним из трёх имеющих атмосферу спутников.
Сатурн
Вторая по размерам планета и шестая по счёту в Солнечной системе. В сравнении с остальными планетами, наиболее схожа с Солнцем составом химических элементов. Радиус поверхности равен 57350 км, год составляет 10 759 суток (почти 30 земных лет). Сутки здесь длятся немногим дольше, чем на Юпитере – 10,5 земных часов. Количеством спутников он ненамного отстал от своего соседа – 62 против 67. Самым крупным спутником Сатурна является Титан, так же, как и Ио, отличающийся наличием атмосферы. Немного меньше него по размеру, но от этого не менее известные – Энцелад, Рея, Диона, Тефия, Япет и Мимас. Именно эти спутники являются объектами для наиболее частого наблюдения, и потому можно сказать, что они наиболее изучены в сравнении с остальными.
Сатурн, снимок космического аппарата Кассини в 2007 году
Долгое время кольца на Сатурне считались уникальным явлением, присущим только ему. Лишь недавно было установлено, что кольца имеются у всех газовых гигантов, но у остальных они не настолько явно видны. Их происхождение до сих пор не установлено, хотя существует несколько гипотез о том, как они появились. Кроме того, совсем недавно было обнаружено, что неким подобием колец обладает и Рея, один из спутников шестой планеты.
Уран
Седьмая по счету и третья по размеру планета, радиус которой составляет 25267 км. Справедливо считается самой холодной планетой среди остальных, температура достигает -224 градусов по Цельсию. Продолжительность года — 30 685 суток в земном исчислении (почти 84 года), сутки же ненамного меньше земных – 17 с небольшим часов. Из-за сильной наклонности оси планеты, иногда создается впечатление, будто она не вращается, как остальные небесные тела нашей системы, а катится, подобно шару. Это может наблюдать любой, кого интересует астрономия, геометрическая модель солнечной системы наглядно продемонстрирует этот эффект.
Уран — снимок Вояджера-2 в 1986 году
Спутников у него гораздо меньше, чем у соседнего Сатурна, всего 27. Наиболее известны Титания, Ариэль, Оберон, Умбриэль и Миранда. Они не настолько крупны, как спутники.
Примечательно, что ведя наблюдения за Ураном в свой телескоп, астроном Уильям Гершель сначала не понял, что он наблюдает за планетой, будучи уверен, что он видит комету.
Нептун
Размером восьмая планета солнечной системы очень близка к своему ближайшему соседу, Урану. Радиус Нептуна равняется 24547 км. Год на планете равняется 60 190 суток (приблизительно 164 земных года). В атмосфере зафиксированы самые сильные ветра в нашей системе, скорость которых достигает 260 м/с.
Нептун, вид с Вояджера-2
По сравнению с остальными планетами-гигантами спутников у него совсем мало – всего 14. Самые известные из них – Тритон, третий в солнечной системе спутник, имеющий атмосферу, Протей и Нереида.
Примечательно, что это – единственная из планет, которая была открыта не благодаря наблюдениям, а с помощью математических расчётов.
Планеты Солнечной системы | |
---|---|
Карликовые планеты | Плутон· Церера· Хаумеа· Макемаке· Эрида |
Планеты Земной группы | Меркурий· Венера· Земля· Марс |
Газовые гиганты | Юпитер· Сатурн· Уран· Нептун |
Самая маленькая и самая внутренняя планета от Солнца в Солнечной системе
![]() | ||||||||||||
Обозначения | ||||||||||||
---|---|---|---|---|---|---|---|---|---|---|---|---|
Произношение | (![]() | |||||||||||
Прилагательные | меркурианец,. Mercurial | |||||||||||
Орбитальные характеристики | ||||||||||||
Эпоха J2000 | ||||||||||||
Афелий |
| |||||||||||
Перигелий |
| |||||||||||
Большая полуось |
| |||||||||||
Эксцентриситет | 0,205630 | |||||||||||
Орбитальный период |
| |||||||||||
Синодический период | 115,88 d | |||||||||||
Средняя орбитальная скорость | 47,362 км / с | |||||||||||
Средняя аномалия | 174,796 ° | |||||||||||
Наклонение <675 От>7,005 ° до эклиптики | ||||||||||||
Долгота восходящего | 48,331 ° | |||||||||||
Аргумент перигелия | 29,124 ° | |||||||||||
Спутники | Нет | |||||||||||
Физические характеристики | ||||||||||||
Средний диаметр | 4880 км | |||||||||||
Средний радиус |
| |||||||||||
Сплющивание | 0,0000 [1 ] | |||||||||||
Площадь поверхности |
| |||||||||||
Объем |
| |||||||||||
Масса |
| |||||||||||
Средняя плотность | 5,427 г / см | |||||||||||
Плотность на поверхности |
| |||||||||||
Момент инерции | 0,346 ± 0,014 | |||||||||||
Скорость убегания | 4,25 км / с | |||||||||||
Сидерический период вращения |
| |||||||||||
Экваториальное вращение скорость | 10,892 км / ч (3,026 м / с) | |||||||||||
Наклон оси | 2,04 ′ ± 0,08 ′ (до орбиты). (0,034 °) | |||||||||||
Северный полюс прямое восхождение |
| |||||||||||
Северный полюс склонение | 6 1,45 ° | |||||||||||
Альбедо |
| |||||||||||
| ||||||||||||
Видимая звездная величина | от -2,48 до +7,25 | |||||||||||
Угловой диаметр | 4,5–13 ″ | |||||||||||
Атмосфера | ||||||||||||
Поверхность давление | след (≲ 0,5 нПа) | |||||||||||
Состав по объему |
| |||||||||||
Меркурий — самая маленькая и самая внутренняя планета в Солнечной Системы. Его орбита вокруг Солнца занимает 87,97 дня, это самая короткая из всех планет Солнечной системы. Он назван в честь греческого бога Гермеса (Ερμής), переведенного на латинский язык: Меркурий Меркурий, бог торговли, посланник богов, посредник между богами и смертными.
Подобно Венере, Меркурий вращается вокруг Солнца в пределах земной орбиты как подчиненная планета, и его видимое расстояние от Солнце, если смотреть с Земли, никогда не больше 28 °. Эта близость к Солнцу означает, что планету можно увидеть около западного горизонта после захода или восточного горизонта до восхода, обычно в сумерках. В этом время он может выглядеть как яркий звездообразный объект, но его часто труднее вести, чем Венеру. Планета телескопически отображает полный диапазон фаз, подобных Венере и Луне, когда она движется по своей внутренней орбите относительно Земли, что повторяется в течение ее синодического периода, составляющего 116 дней..
Меркурий вращается уникальным образом в Солнечной системе. Он приливно синхронизирован с Солнцем в спин-орбитальном резонансе 3: 2 , что означает, что относительно неподвижных он вращается вокруг своей оси ровно на три раз за каждые два оборота вокруг Солнца. Как видно с Солнца, в системе отсчета , которая вращается вместе с орбитальным движением, кажется, что оно вращается вместе каждые два меркурианских года. Следовательно, наблюдатель на Меркурии будет видеть только один день каждые два меркурианских года.
Ось Меркурия имеет наименьший наклон любой из планет Солнечной системы (около ⁄ 30 градусов). Его эксцентриситет орбиты — самый большой из всех известных планет Солнечной системы; в перигелии расстояние от Меркурия до Солнца составляет лишь около двух третей (или 66%) его расстояния в афелии. Поверхность Меркурия сильно испещрена кратерами и похожа на поверхность Луны, что указывает на то, что она была геологически неактивной в течение миллиардов лет. В нем почти нет атмосферы для сохранения тепла, а температура поверхности его поверхности в течение суток меняется сильнее, чем на любой другой планете Солнечной системы, от 100 К (-173 ° C; -280 ° F) ночью до 700 K (427 ° C)). ; 800 ° F) в течение дня в экваториальных регионах. В полярных регионах постоянно ниже 180 К (-93 ° C; -136 ° F). На планете известных естественных спутников.
Два космических корабля используют Меркурий: Mariner 10 пролетел в 1974 и 1975 годах; и MESSENGER, запущенный в 2004 году, совершил оборот вокруг Меркурия более 4000 раз за четыре года, прежде чем израсходовать топливо и врезаться на поверхность планеты 30 апреля 2015 года. Планируется прибытие космического корабля BepiColombo на Меркурии в 2025 году.
Содержание
- 1 Физические характеристики
- 1.1 Внутренняя структура
- 1.2 Геология поверхности
- 1.2.1 Ударные бассейны и кратеры
- 1.2.2 Равнины
- 1.2.3 Компрессионные характеристики
- 1.2.4 Вулканология
- 1.3 Состояние поверхности и экзосфера
- 1.4 Магнитное поле и магнитосфера
- 2 Орбита, вращение и долгота
- 2.1 Условные обозначения долготы
- 2.2 Спин-орбитальный резонанс
- 2.3 Развитие перигелия
- 3 Биологические соображения
- 3.1 Пригодность
- 4 Наблюдение
- 5 История наблюдений
- 5.1 Древние астрономы
- 5.2 Наземные телескопические исследования
- 5.3 Исследования в космосе зонды
- 5.3.1 Mariner 10
- 5.3.2 MESSENGER
- 5.3.3 BepiColombo
- 6 Сравнение
- 7 См. также
- 8 Примечания
- 9 Ссылки
- 10 Внешние ссылки
Физические характеристики
Внутренняя структура
Внутренняя структура и магнитное поле Меркурия
Похоже, что Меркурий имеет твердую силикатную корку и мантию, покрывающую твердый внешний слой ядра из сульфида железа, более глубокую жидкость ядра и твердое внутреннее ядро.
Меркурий является одной из четырех планет земной группы в Солнечной системе и представляет собой твердое тело, подобное Земле. Это самая маленькая планета в Солнечной системе с экваториальным радиусом 2439,7 км (1516,0 миль). Меркурий также меньше — хотя и более массивен — чем самые большие естественные спутники в Солнечной системе, Ганимед и Титан. Ртуть состоит из 70% металлического и 30% силикатного материала. Плотность Меркурия является второй по величине в Солнечной системе и составляет 5 427 г / см, что лишь немного меньше плотности Земли, равной 5 515 г / см. Если бы эффект гравитационного сжатия был исключен из планет, материалов, из которых были бы плотнее, чем у Земли, с плотностью без сжатия 5,3 г / см по сравнению с плотностью Земли 4,4 г / см. см.
Плотность Меркурия может Роман, чтобы сделать вывод о деталях его внутренней структуры. Хотя высокая плотность Земли в области степени является результатом гравитационного сжатия, особенно в ядре , Меркурий намного меньше, и его внутренние не так сжаты. Следовательно, чтобы иметь такую высокую плотность.
По оценкам геологов, ядро ядро занимает около 55% его объема Меркурия; для Земли эта доля составляет 17%. Исследование опубликованное в 2007 году предполагает, что у Меркурия есть расплавленное ядро. Ядро окружает 500–700 км (310–430 миль) мантия, состоящая из силикатов. Основываясь на данных миссии Mariner 10 и наземных наблюдений, толщина коры Меркурия оценивается в 35 км (22 мили). Отличительной особенностью поверхности Меркурия является наличием множества узких хребтов, протянувшихся до нескольких сотен километров в длину. Считается, что они образовались, когда ядро и мантия Меркурия охлаждались и сжимались в то время, когда кора уже затвердела.
Ядро Меркурия имеет более высокое содержание железа, чем у любой другой крупной планеты Солнечной системы. и для объяснения этого было предложено несколько теорий. Наиболее широко распространенная теория состоит в том, что Меркурий имеет соотношение металл / силикат, обычное хондритовым метеоритам, которые считаются типичными для каменистого вещества Солнечной системы, а его масса примерно в 2,25 раза больше ее нынешней массы. В начале истории Солнечной системы Меркурий, возможно, был поражен планетезималью массой примерно 1/6 этой массы и диаметром в несколько тысяч километров. Удар снес бы большую часть первоначальной коры и мантии. Подобный процесс, известный как гипотеза гигантского удара, был предложен для объяснения образования Луны.
. В качестве альтернативы, Меркурий мог образоваться из солнечной туманности раньше. выход энергии Солнца стабилизировался. Первоначально он имел бы вдвое большую массу, чем сейчас, но по мере того, как протосолнце сжималось, температура около Меркурия могла быть между 2500 и 3500 К и, возможно, даже до 10000 К. Большая часть поверхностных пород Меркурия могла быть испаряется при таких температурах, образуя среду «каменного пара», который мог быть унесен солнечным ветром.
Третья гипотеза предполагает, что солнечная туманность вызвала сопротивление на материалах, от которых Меркурий аккрецировал, что означало, что более легкие частицы были потеряны из аккрецирующего материала, а не собраны Меркурием. Каждая гипотеза предсказывает различный состав поверхности, и есть две космические миссии для проведения наблюдений. MESSENGER, завершившаяся в 2015 году, обнаружила более высокие, чем ожидалось, уровни калия и серы на поверхности, предполагая, что гипотеза гигантского удара и испарение коры и мантии не произошло, потому что калий и сера были из-за сильной жары этих событий. БепиКоломбо, который прибудет к Меркурию в 2025 году, проведет наблюдения, чтобы проверить эти гипотезы. Полученные к настоящему моменту результаты подтверждают третью гипотезу; однако необходим дальнейший анализ данных.
Геология поверхности
Топографическая карта северного полушария Меркурия, полученная прибором MLA на MESSENGER. самый низком (фиолетовый) на самый высокий (красный, 10 км) (6,2 мили)).
Топографическая карта Меркурия
Поверхность Меркурия похожа на поверхность Луны, действующие морские равнины и тяжелый кратер, указывающий на то, что он был геологически неактивным в течение нескольких лет. Знания о геологии Меркурия были основаны только на пролетах Маринера 10 1975 года и наблюдениях за землей, это названиеее изученная из планет земной группы. По мере обработки данных с орбитального аппарата MESSENGER эти знания будут увеличиваться. Например, обнаружен необычный кратер с расходящимися впадинами, который ученые назвали «пауком». Позже он был назван Аполлодор.
Поверхность Меркурия
MASCS сканирование поверхности Меркурия по MESSENGER
Альбедо. Особенности — это области с заметно различающейся отражательной способностью, как видно из телескопических наблюдений.. У Меркурия есть дорса (также называемая «морщинистые гребни »), луноподобные нагорья, горы (горы), планитии (равнины), рупы (откосы) и долины. (valleys).
Имена функций на Меркурии взяты из разных источников. Имена, исходящие от людей, ограничиваются умершими. Кратеры названы в честь художников, музыкантов, художников и авторов, которые внесли выдающийся или фундаментальный вклад в свою область. Хребты, или дорса, названы в честь ученых, внесших вклад в изучение Меркурия. Впадины или ямки названы в честь произведений архитектуры. Монтес назван в честь слова «горячий» на разных языках. Равнины или planitiae названы в честь Меркурия на разных языках. Откосы или рупес названы в честь кораблей научных экспедиций. Долины или долины названы в честь заброшенных городов, поселков или поселений древности.
Меркурий подвергся сильной бомбардировке комет и астероидов во время и вскоре после его образования 4,6 миллиарда лет назад, а также во время, возможно, отдельного последующего эпизода, названного поздней тяжелой бомбардировкой, который закончился 3,8 миллиарда лет назад. В течение этого периода интенсивного кратерообразования Меркурий получил удары по всей своей поверхности, способствовало отсутствию какой-либо атмосферы, которая могла бы замедлить ударные волны. В это время Меркурий был вулканически активным; бассейны, такие как Бассейн Калорис, были заполнены магмой, образуя гладкие равнины, подобные морям, найденным на Луне.
Данные из Облет MESSENGER в октябре 2008 года исследователям большее представление о беспорядочной природе поверхности Меркурия. Поверхность Меркурия более неоднородна, чем поверхность Марса или Луны, оба из которых содержат основные участки схожей геологии, такие как моря и плато.
Ударные бассейны и кратеры
Перспективный вид бассейна Калорис — высокий (красный); низкий (синий).
Цветное изображение кратеров Мунк, Сандер и По среди вулканических равнин (оранжевый) около Бассейна Калорис
Диаметр кратеров на Меркурии изменяется от небольших полостей в форме чаши до многокольцевые ударные бассейны в поперечнике. Они появляются во всех состояниях деградации, от относительно свежих лучевых кратеров до сильно разрушенных остатков кратеров. Кратеры Меркурия незначительно отличаются от лунных кратеров тем, что область, покрытая их выбросами, намного меньше, чем они являются следствием более сильной поверхностной гравитации Меркурия. Согласно правилам IAU, каждый новый кратер должен быть назван в честь художника, который известен более пятидесяти лет и умер более трех лет, до того, как будет назван кратер.
Самый большой известный кратер — Бассейн Калорис, диаметром 1 550 км. Удар, создавший Бассейн Калорис, был настолько мощным, что вызвал извержения лавы и оставил концентрическое кольцо высотой более 2 км, окружающее ударный кратер . В антиподе Бассейна Калорис находится большой регион необычной холмистой местности, известный как «Странная местность». Одна из гипотез его происхождения заключается в том, что ударные волны, образовавшиеся во время удара Калориса, прошли вокруг Меркурия, сход в антиподе бассейна (на 180 градусов). В результате высокого напряжения разрушили поверхность. В качестве альтернативы было высказано предположение, что эта местность образована в результате схождения выбросов этого антиподе.
В целом, на изображенной части Меркурия было идентифицировано около 15 ударных бассейнов. Примечательная впадина является многокольцевая котловина Толстого шириной 400 км, у которой есть выброс из выброса, простирающийся до 500 км от ее края, и дно, заполненное гладкими равнинными материалами. Бассейн Бетховена имеет аналогичное по размеру одеяло выброса и край диаметром 625 км. Как и Луна, поверхность Меркурия, вероятно, подверглась воздействию космического выветривания, включая удары солнечного ветра и микрометеорита.
Внутренняя часть кратера Абедин
Равнины
Бассейн Калорис, один из систем ударных бассейнов в Солнечной системе
Так называемая «Странная местность» образовалась в точке противоположной точки до удара Бассейна Калорис.
На Меркурии есть две геологически разные равнинные области. Плавные холмистые равнины в областях между кратерами — самые старые видимые поверхности Меркурия, предшествующие сильно изрезанной кратерами местности. Эти межкратерные равнины, по-видимому, стерли лица земли более ранние кратеры, и в целом наблюдается небольшое количество меньших кратеров диаметром менее 30 км.
Гладкие равнины — это широко распространенные плоские области, которые заполняют депрессии различных размеров и несут на себе сильную поверхность. сходство с лунной марией. Примечательно, что они заполняют широкое кольцо, которое Бассейн Калорис. В отличие от лунных морей, гладкие равнины Меркурия имеют такое же альбедо, что и более старые равнины между кратерами. Несмотря на отсутствие однозначно вулканических характеристик, расположение и округлая, лопастная форма этих равнин убедительно свидетельствует о вулканическом происхождении. Все гладкие равнины Меркурия сформировались позже бассейна Калорис, о чем свидетельствуют заметно меньшие плотности кратеров, чем на бланкете выброса Калорис. Дно бассейна Калорис заполнено геологически отчетливой плоской равниной, разбитой гребнями и трещинами в виде примерно многоугольной структуры. Неясно, являются ли они вулканическими лавами, образовавшимися в результате удара, или большим слоем ударного расплава.
Особенности сжатия
Одной из необычных особенностей поверхности Меркурия являются многочисленные складки сжатия или руп, пересекающие равнины. Когда внутреннее пространство Меркурия остыло, оно сжималось, и его поверхность начала деформироваться, создавая морщинистые гребни и лопастные уступы, связанные с надвигами. Переходы могут достигать длины 1000 км и высоты до 3 км. Эти детали сжатия можно увидеть поверх других деталей, таких как кратеры и гладкие равнины, что указывает на то, что они появились позже. Картирование особенностей предполагает полное сокращение радиуса Меркурия в диапазоне от ~ 1 до 7 км. Обнаружены мелкомасштабные уступы надвиговых разломов высотой в несколько десятков метров и длиной в диапазоне нескольких километров, возраст которых, по всей видимости, составляет менее 50 миллионов лет, что указывает на то, что сжатие внутренних поверхностей и последующая геологическая активность на поверхности продолжают усиливаться. настоящее.
Лунный разведывательный орбитальный аппарат обнаружил, что аналогичные небольшие надвиги существуют на Луне.
Вулканология
кратер Пикассо — большой дугообразный Яма, расположенная на восточной стороне ее дна, предположительно образовалась, когда подземная магма опустилась или осушилась, что привело к обрушению поверхности в образовавшуюся пустоту.
Изображения, полученные MESSENGER, выявили доказательства пирокластических потоков на Меркурии из низкопрофильных щитовых вулканов. Данные MESSENGER помогли идентифицировать 51 пирокластическое отложение на поверхности, 90% из которых находятся в ударных кратерах. Изучение состояния деградации ударных кратеров, вмещающих пирокластические отложения, предполагает, что пирокластическая активность происходила на Меркурии в течение длительного интервала времени.
«Безободковая впадина» внутри юго-западной кромки бассейна Калорис состоит как минимум из девяти перекрывающих друг друга вулканических жерл, каждый диаметром до 8 км каждый. Таким образом, это «составнойвулкан ». Днища вентиляционных отверстий находятся как минимум на 1 км ниже их краев, и они больше похожи на вулканические кратеры, образованные взрывными извержениями или преобразованные в результате обрушения пустоты, образованные отводом магмы обратно в канал. Ученые не смогли определить возраст вулканической сложной системы, но сообщили, что он может быть миллиарда лет.
Состояние поверхности и экзосфера
Составное изображение Северного полюса Меркурия, полученное с помощью MESSENGER
Radar изображение северного полюса которого Меркурия
Составное изображение северного полюса Меркурия, наСА подтвердило открытие большого объема водяного льда в постоянно темных кратерах, обнаруженных там.
Температура поверхности Меркурия колеблется от 100 до 700 K (от −173 до 427 ° C; от −280 до 800 ° F) в самых экстремальных местах: 0 ° N, 0 ° W или 180 ° W. выше 180 К на полюсах из-за атмосферы и крутого температурного градиента между экватором и полюсами. Подсолнечная точка достигает около 700 К во время перигелия (0 ° з.д. или 180 ° з.д.), но только 550 К в афелии (90 ° или 270 ° з.д.). На темной стороне планеты средняя температура составляет 110 К. Интенсивность солнечного света на поверхности Меркурия колеблется от 4,59 до 10,61 раз от солнечной постоянной (1370 Вт · м).
Хотя дневная температура на поверхности Меркурия обычно высока, наблюдения показывают, что на Мерку существует лед (замороженная вода). Дно глубоких кратеров на полюсах не попадает под прямые солнечные лучи, и температура там остается ниже 102 К; намного ниже, чем в среднем по миру. Водяной лед сильно отражает радар, и наблюдения с помощью 70-метрового радара солнечной системы Голдстоуна и VLA в начале 1990-х годов показали, что есть участки высокого радара. отражение у полюсов. Хотя лед был не единственной возможной появления этих отражающих областей, астрономы считают, что это наиболее вероятно.
Ледяные области, по оценкам, содержат около 10–10 кг льда и могут быть покрыты слоем льда. реголит, ингибирующий сублимацию. Для сравнения: антарктический ледяной щит на Земле имеет массу около 4 × 10 кг, а южная полярная шапка Марса содержит около 10 кг воды. Происхождение льда на Мерку еще не известно, но два наиболее вероятных источника — это выделение газа из недр планеты или отложение в результате ударов комет.
Меркурий слишком мал и горячий из-за своей гравитации, чтобы удерживать значительную атмосферу в течение длительных периодов времени; он имеет тонкую ограниченную поверхность экзосферу, содержащую водород, гелий, кислород, натрий, кальций, калий и другие при поверхностном давлении менее примерно 0,5 нПа (0,005 пикобар). Эта экзосфера нестабильна — атомы теряются и пополняются постоянно из различных источников. Атомы водорода и атомы гелия, вероятно, происходит из солнечного ветра, диффундируют в магнитосферу Меркурия, прежде чем позже уйти обратно в космос. Радиоактивный распад элементов в коре Меркурия — еще один источник гелия, а также натрия и калия. MESSENGER обнаружил большое количество кальция, гелия, гидроксида, магния, кислорода, калия, кремния и натрия. Присутствует водяной пар, выделяемый комбинацией процессов, таких как: удары комет по его поверхности, распыление, создание воды из водорода солнечного ветра и кислорода из горных пород, а также сублимация из резервуаров. водяного льда в постоянно затененных полярных кратерах. Обнаружение такого количества связанных с водой автомобилей, как O, OH и H3O, стало неожиданностью. Исследователи предполагают, что эти молекулы выброшены с поверхности или экзосферы солнечным ветром.
Натрий, калий и кальций были обнаружены в атмосфере во время 1980–1990-е годы, и считается, что это в первую очередь результат испарения поверхностных пород в результате ударов микрометеоритов, в том числе в настоящее время от кометы Энке. В 2008 году MESSENGER открыл магний. Исследования показывают, что иногда выбросы натрия в точках, соответствующих магнитным полюсам планеты. Это указывало бы на взаимодействие между магнитосферой и поверхностью планеты.
29 ноября 2012 года НАСА подтвердило, что изображения с MESSENGER представили, что кратеры на северном полюсе содержат водяной лед. Главный исследователь MESSENGER Шон Соломон цитируется в The New York Times, оценивая объем льда как достаточно большой, чтобы заключить Вашингтон, округ Колумбия, в замороженный блок на две с половиной мили. deep «.
Магнитное поле и магнитосфера
График, показывающий относительную силу магнитного поля Меркурия
, несмотря на свой небольшой размер и медленное 59-дневное вращение, Меркурий имеет значительную и, по-предположительно, глобальную магнитное поле. Согласно измерениям, проведенным Mariner 10, это примерно 1,1% прочности Земли. Напряженность магнитного поля на экваторе Меркурия составляет около 300 нТл. 227>диполярное. В отличие от Земли, полюса Меркурия почти совпадают с осью планеты. Измерения космических зондов Mariner 10 и MESSENGER показали, что сила и форма магнитного поля стабильны.
Вероятно, это магнитное поле создается. эффектом динамо, аналогично магнитному полю Земли. Этот динамо-эффект был бы циркуляции богатого железом жидкого ядра планеты. особенно сильные приливные эффекты, вызванные большим эксцентриситетом орбиты планеты, будут поддержанию ядра в жидком состоянии, необходимом для этого динамо-эффект.
Магнитное поле Меркурия достаточно сильное, чтобы отклонять солнечный ветер вокруг планеты, создавая магнитосферу. Магнитосфера планеты, хотя и достаточно мала, чтобы поместиться в пределах Земли, достаточно сильна, чтобы улавливать солнечный ветер плазму. Это способствует космическому выветриванию поверхности планеты. Наблюдения, проведенные космическим кораблем Маринер-10, представьте эту низкоэнергетическую плазму в магнитосфере на ночной стороне планеты. Всплески энергичных частиц в хвосте магнитосферы указывают на динамическое качество магнитосферы планеты.
Во время второго пролета над планетой 6 октября 2008 года МЕССЕНДЖЕР обнаружил, что магнитное поле Меркурия может быть очень «проникающим». Космический корабль столкнулся с магнитными «торнадо» — скрученными пучками магнитных полей, соединяющих магнитное поле планеты с межпланетным пространством, шириной до 800 км, или одной трети радиуса планеты. Эти закрученные трубки магнитного потока, технически известные как события передачи потока, образуют открытые окна в магнитном щите планеты, через солнечный ветер может проникать и воздействовать напрямую на поверхность Меркурия посредством магнитного пересоединения. происходит в магнитном поле Земли. Наблюдения МЕССЕНДЖЕР показал, что скорость пересечения у Меркурия десять раз выше, но его близость к Солнцу составляет около трети скорости пересоединения, наблюдаемой МЕССЕНДЖЕР.
Орбита, вращение и долгота
Орбита Меркурия (2006)
Анимация вращения Меркурия и Земли вокруг Солнца
У Меркурия самая эксцентрическая орбита из всех планет; его эксцентриситет составляет 0,21, расстояние от Солнца составляет от 46 000 000 до 70 000 000 км (от 29 000 000 до 43 000 000 миль). Полный оборот по орбите занимает 87 969 земных суток. Диаграмма показывает эффекты эксцентриситета, форму орбиту Меркурия, наложенную на круговую орбиту, имеющую ту же самую большую полуось . Более высокая скорость Меркурия, когда он находится около перигелия, очевидна с большего расстояния, которое он совершает через каждый 5-дневный интервал. На диаграмме изменяющееся расстояние от Меркурия до Солнца представлено размером планеты, который обратно пропорционально расстоянию до Солнца. Такое расстояние до Солнца приводит к изменению поверхности Меркурия за счет приливных выпуклостей, поднимаемых Солнцем, которые примерно в 17 раз сильнее, чем у Луны на Земле. В сочетании со спин-орбитальным резонансом 3: 2 вращения вокруг своей оси это также приводит к сложным изменениям температуры поверхности. Резонанс заставляет один солнечный день на Меркурии длиться ровно два года по Меркурию, или около 176 земных дней.
Орбита Меркурия наклонена на 7 градусов к плоскости орбиты Земли (эклиптика ), как показано на диаграмме справа. В результате прохождение Меркурия через поверхность Солнца может происходить только тогда, когда планета пересекает плоскость эклиптики в то время, когда она находится между Землей и Солнцем, то есть в мае или ноябре. Это происходит в среднем каждые семь лет.
Наклон оси Меркурия почти равенство нулю, а наилучшее измеренное значение составляет 0,027 градуса. Это значительно меньше, чем у Юпитера, который имеет второй по величине наклон оси среди всех планет на 3,1 градуса. Это означает, что для наблюдателя на полюсах Меркурия центр Солнца поднимается над горизонтом более чем на 2,1 угловых минут.
В некоторых точках на поверхности Меркурия наблюдатель можно увидеть, как Солнце выглядит немного больше, чем на две трети пути над горизонтом, и все это снова в пределах того же самого самого меркурианского дня. Это связано с тем, что примерно за четыре земных дня до перигелия угловая орбитальная скорость Меркурия равна его угловой скорости вращения, так что видимое движение Солнца прекращается. ; ближе к перигелию угловая орбитальная скорость Меркурия превышает угловую скорость вращения. Таким образом, гипотетическим наблюдателем на Меркурии кажется, что Солнце движется в ретроградном направлении. Через четыре земных дня после перигелия возобновляется нормальное видимое движение Солнца. Аналогичный эффект произошел бы, если бы Меркурий находился в синхронном вращении: чередование усиления и произошло бы к либрации 23,65 ° по долготе.
По той же причине есть две точки на Экватор Меркурия, разнесенный на 180 градусов на долготе, в любом из которых, вокруг перигелия в чередующиеся меркурианские годы (один раз в меркурианские дни), Солнце проходит над головой, затем меняет свое видимое движение и снова проходит над головой, затем меняет направление. во второй раз и проходит над головой в сумме занимает около 16 земных дней для всего этого процесса. В другие альтернативные меркурианские годы то же самое происходит в другой из этих двух точек. Ампли ретроградного движения мала, поэтому в течение общего эффекта в том, что в двух или трех недель Солнце почти неподвижно над головой и является наиболее ярким, потому что Меркурий находится в перигелии, ближайшем к Солнцу. Это продолжительное пребывание на Солнце с максимальной яркостью делает эти две точки самыми горячими местами на Меркурии. Максимальная температура, когда Солнце находится под примерно углом 25 градусов после полудня из-за дневного температурного лага, на 0,4 Меркурийных дня и 0,8 Меркурийских лет после восхода Солнца. И наоборот, на экваторе есть две другие точки, удаленные от первых на 90 градусов долготы, где Солнце проходит над головой только тогда, когда планета находится в афелии в разные годы, когда появляется видимое движение Солнца в небе Меркурия относительно быстро.. Эти точки на экваторе, где происходит очевидное ретроградное движение Солнца, когда оно пересекает горизонт, как показано в предыдущем абзаце, получают меньше намного солнечного тепла, чем первые, описанные выше.
Меркурий достигает нижнего соединения (ближайшего сближения с Землей) в среднем каждые 116 земных дней, но этот интервал может варьироваться от 105 до 129 дней из-за эксцентрической орбиты планеты. Меркурий может приблизиться к Земле на расстояние 82,2 гига метра (0,549 астрономических единиц; 51,1 миллиона миль), и это медленно уменьшается: следующий подход с точностью до 82,1 Gm (51,0 миллиона миль) — это 2679, а в пределах 82,0 Gm (51,0 миллиона миль). миль) в 4487, но он не будет ближе к Земле, чем 80 Gm (50 миллионов миль) до 28 622. Его период ретроградного движения при наблюдении с Земли может варьироваться от 8 до 15 дней по обе стороны от нижнего соединения. Этот большой диапазон возникает из-за большого эксцентриситета орбиты планеты. В среднем Меркурий — ближайшая планета к Земле и самая близкая планета к каждой из других планет Солнечной системы.
Условное обозначение долготы
Условное обозначение долготы для Меркурия помещает ноль долготы в одной из двух самых горячих точек на поверхности, как описано выше. Однако, когда Mariner 10 впервые посетил эту область, этот нулевой меридиан был в темноте, поэтому было невозможно выбрать объект на поверхности, чтобы определить точное положение меридиана. Поэтому был выбран небольшой кратер, расположенный дальше на запад, под названием Хун Кал, который является точной точкой отсчета для измерения долготы. Центр Хун Кала определяет 20 ° западного меридиана. Резолюция Международного астрономического союза 1970 года предполагает, что долготы измеряются положительно в западном направлении на Меркурии. Таким образом, два самых жарких места на экваторе находятся на долготе 0 ° W и 180 ° W, а самые холодные точки на экваторе — на долготе 90 ° W и 270 ° W. Однако в проекте MESSENGER используется восточно-положительное соглашение.
Спин-орбитальный резонанс
После одной орбиты Меркурий повернулся в 1,5 раза, поэтому после двух полных оборотов снова засветилось одно и то же полушарие.
В течение многих лет считалось, что Меркурий был синхронно приливно заблокирован с Солнцем, вращаясь один раз для каждой орбиты и всегда сохраняя одну и ту же грань, направленную к Солнцу, точно так же, как всегда обращена одна и та же сторона Луны. Земля. Радарные наблюдения в 1965 году доказали, что планета имеет спин-орбитальный резонанс 3: 2, вращаясь три раза за каждые два оборота вокруг t он вс. Эксцентриситет орбиты Меркурия делает этот резонанс стабильным — в перигелии, когда солнечный прилив наиболее сильный, Солнце почти все еще находится в небе Меркурия.
Редкий резонансный приливный захват 3: 2 стабилизируется дисперсией приливной силы вдоль эксцентрической орбиты Меркурия, действующая на постоянную дипольную составляющую распределения массы Меркурия. На круговой орбите такой дисперсии нет, поэтому единственный стабилизированный на такой орбите резонанс составляет 1: 1 (например, Земля — Луна), когда приливная сила, растягивающая тело вдоль линии «центр-тело», действующий момент, который выравнивает ось тела с наименьшей инерцией («самая длинная» ось и ось вышеупомянутого диполя) так, чтобы она всегда указывала в центре. Однако с помощью эксцентриситетом, как у орбиты Меркурия, приливная сила имеет максимум в перигелии и поэтому стабилизирует резонансы, такие как 3: 2, заставляя планету направлять свою ось наименьшей инерции примерно на Солнце при прохождении перигелия.
Первоначальная причина, по которой астрономы считали, что он синхронизирован, заключалась в том, что всякий раз, когда Меркурий был лучше всего расположен для наблюдения, он всегда был почти в одной и той же точке в своем резонансе 3: 2, следовательно, созданное одно и то же лицо. Это потому, что по совпадению, период вращения Меркурия составляет почти ровно половину его синодического периода по отношению к земле. Из-за спин-орбитального резонанса 3: 2 Меркурия солнечный день (длина между двумя меридианом транзитами Солнца) длится около 176 земных дней. сидерический день (период вращения) длится около 58,7 земных суток.
Моделирование показывает, что орбитальный эксцентриситет Меркурия хаотически изменяется от почти нуля (круговой) до более чем 0,45 за миллионы лет из-за возмущений со стороны других планет. Считалось, что это объясняет спин-орбитальный резонанс 3: 2 Меркурия (а не более обычный 1: 1), поскольку это состояние с большей вероятностью возникнет в период высокого эксцентриситета. Однако точное моделирование, основанное на реалистичной модели приливной реакции, применимо, что Меркурий был закреплен в спинномозговой орбиты 3: 2 на очень ранней стадии своей истории, в течение 20 (более вероятно, 10) миллионов лет после своего образования.
Численное моделирование показывает, что будущее световое орбитальное резонансное взаимодействие перигелия с Юпитером может привести к увеличению эксцентриситета орбиты Меркурия до точки, при которой существует вероятность 1%. что планета столкнется с Венерой в течение следующих пяти миллиардов лет.
Продвижение перигелия
В 1859 году французский математик и астроном Урбен Леверье сообщил, что медленный прецессия орбиты Меркурия вокруг Солнца не может быть полностью объяснена механикой Ньютона и возмущениями со стороны известных планет. Он предположил, среди объяснений, что другая планета (или, возможно, вместо этой серии меньших «корпускул») могла существовать на орбите, даже более близкой к Солнцу, чем Меркурий, чтобы это возмущение. (Среди других рассмотренных объяснений было небольшое сжатие Солнца.) Успех поиска Нептуна на основе его возмущений орбиты Урана заставил астрономов поверить в это возможное объяснение., и гипотетическая планета была названа Вулкан, но такая планета никогда не была найдена.
прецессия перигелия Меркурия составляет 5600 угловых секунд (1,5556 °) на столетие относительно Земли, или 574,10 ± 0,65 угловых секунд на столетие относительно инерционного МКРФ. Механика Ньютона, учитывая все эффекты от других планет, предсказывает прецессию в 5,557 угловых секунд (1,5436 °) за столетие. В начале 20 века общая теория относительности Альберта Эйнштейна предоставила объяснение наблюдаемой прецессии, формализовав гравитацию как опосредованную кривизной пространства-времени. Эффект невелик: всего 42,98 угловых секунды в столетие для Меркурия; поэтому для полного избыточного витка требуется немногим более двенадцати миллионов витков. Подобные, но гораздо меньшие эффекты существуют для других тел Солнечной системы: 8,62 угловых секунды в столетие Венеры, 3,84 для Земли, 1,35 для Марса и 10,05 для 1566 Икар.
Формула Эйнштейна для с Нарушение перигелия за оборот: ϵ знак равно 24 π 3 a 2 T 2 c 2 (1 — e 2) { displaystyle epsilon = 24 pi ^ {3} { frac {a ^ {2}} { T ^ {2} c ^ {2} (1-e ^ {2})}}}, где e { displaystyle e}
— орбитальный эксцентриситет, a { displaystyle a}
большая полуось и T { displaystyle T}
орбитальный период. Заполнение значений дает результат 0,1035 угловой секунды на оборот или 0,4297 угловой секунды на земной год, то есть 42,97 угловой секунды на столетие. Это полностью согласуется с принятым перигелия Меркурия в 42,98 угловых секунды за столетие.
Биологические соображения
Пригодность
Может иметь место научное подтверждение, основанное на опубликованных исследованиях. в марте 2020 года за счет того, что части планеты Меркурий мог быть обитаемыми, и, возможно, что жизни, хотя, вероятно, примитивные микроорганизмы, могли существовать на
Наблюдение
Мозаика изображений, сделанная Mariner 10, 1974 г.
кажущаяся величина Меркурия, по расчетам, колеблется в пределах -2,48 (ярче, чем Сириус ) вокруг верхних соединений и +7.25 (ниже предела видимости невооруженным глазом) вокруг нижнего соединения. Средняя видимая величина составляет 0,23, а стандартное отклонение 1,78 — самое большое для любой планеты. Средняя видимая величина в верхнем соединении составляет -1,89, а в нижнем соединении +5,93. Наблюдение за Меркуриемомняется его близостью к Солнцу, поскольку большую часть времени он теряется в солнечном свете. Меркурий можно наблюдать только в течение короткого периода в утренних или вечерних сумерках.
Меркурий, как и несколько других планет и самых ярких звезд, можно увидеть во время полного солнечного затмения.
Как и Луна и Венера, Меркурий демонстрирует фазы, если смотреть с Земли. Он «новый» в нижнем соединении и «полный» в верхнем соединении. Планета становится невидимой с Земли в обоих случаях из-за того, что она закрыта Солнцем, за исключением его новой фазы во время транзита.
Меркурий технически является самым ярким, если смотреть с Земли, когда он находится в полной фазе. Хотя Меркурий находится дальше всего от Земли, когда он заполнен, большая освещенная область, которая видна, и всплеск яркости оппозиции более чем компенсирует расстояние. Верно для Венеры, которая кажется самой яркой, когда это полумесяц, потому что она намного ближе к Земле, чем когда gibbous.
Карта ложных цветов, показывающая максимальные температуры на северном полюсе. region
Тем не менее, самое яркое (полнофазное) появление Меркурия — это невозможное время для практических наблюдений из-за крайней точки Солнца. Меркурий лучше всего вести в первой и последней четверти, хотя это уменьшение яркости. Фазы первой и последней четверти происходят при наибольшем удлинении к востоку и западу от Солнца, соответственно. В обоих случаях между Меркурием и Солнцем колеблется от 17,9 ° в перигелии до 27,8 ° в афелии. При наибольшем западном удлинении Меркурий восходит раньше всего перед восходом солнца, а при наибольшем восточном удлинении он заходит не позднее, чем после захода солнца.
Меркурий можно легко увидеть из тропиков и субтропиков больше, чем из более высоких широт. Если смотреть с низких широт и в нужное время года, эклиптика пересекает горизонт под крутым углом. Меркурий находится на 10 ° над горизонтом, когда планета появляется прямо над Солнцем (т. Е. Его орбита кажется вертикальной) и имеет максимальное удлинение от Солнца (28 °), а также когда Солнце находится на 18 ° ниже горизонта, поэтому небо просто совершенно темно. Этот угол представляет собой максимальную высоту, на которой Меркурий виден в полностью темном небе.
Изображение тектонического рельефа Карнеги-Рупс в искусственных цветах — возвышенность (красный); низкий (синий).
На средних широтах Меркурий чаще и легче виден из Южного полушария, чем из Северного. Это максимальное восточное удлинение происходит только в конце зимы в Южном полушарии. В обоих этих случаях угол, под которым орбита планеты пересекает горизонт, максимален, что позволяет ей подниматься до нескольких часов до восхода солнца в первом случае и не происходит до нескольких часов после захода солнца во втором из средних широт, таких как Аргентина и Южная Африка.
Альтернативный метод наблюдения за Меркурием включает наблюдение за планетой в дневное время, когда условия ясны, в идеале, когда она находится в наибольшем удлинении. Это позволяет легко найти планету даже при использовании телескопов с апертурой 8 см (3,1 дюйма). Необходимо следить за тем, чтобы инструмент не был направлен прямо на Солнце из-за риска повреждений глаз. Этот метод позволяет обойти ограничение на наблюдение сумерек, когда эклиптика установлена на небольшой высоте (например, осенними вечерами).
Наблюдения Меркурия с помощью наземного телескопа показывают только освещенный частичный диск с ограниченными деталями. Первым из двух космических кораблей , посетивших планету, был Mariner 10, который нанес на карту около 45% ее поверхности с 1974 по 1975 год. Второй — космический корабль MESSENGER, который после трех облетов Меркурия в период с 2008 по 2009 год вышел на орбиту вокруг Меркурия 17 марта 2011 года, чтобы изучить нанести на карту остальную часть планеты.
Космический телескоп Хаббла вообще не может вести Меркурий по соображениям безопасности, предотвращающие его наведение слишком близко к Солнцу.
сдвиг на 0,15 оборота за год составляет семилетний цикл (0,15 × 7 ≈ 1,0), на седьмом году Меркурий почти точно (на 7 дней раньше) последовательность явлений, которые он показал семь лет назад.
История наблюдений
Древние астрономы
Меркурий из Liber Astronomiae, 1550 г.
Самые ранние известные записанные наблюдения Ртуть из таблицчек Мул.Апин. Эти наблюдения, скорее всего, были сделаны одним ассирийским астрономом около 14 века до нашей эры. клинопись имя, используемое для обозначения Меркурия на табличках Mul.Apin, транскрибируется как Udu.Idim.Gu u 4.Ud («прыгающая планета»). вавилонский. записи о Меркурии к 1-му тысячелетию до нашей эры. вавилоняне назвали планету Набу в посланника богам в своей мифологии.
Древние знали Меркурий под разными именами в зависимости от того, была ли это вечерняя звезда или Утренняя звезда. Примерно к 350 г. до н.э. древние греки осознал, что две звезды — это одна. Они знали планету как Στίλβων Stilbōn, что означает «мерцание», и Ἑρμής Hermēs за ее мимолетное движение — название, сохранившееся в современном греческом языке (Ερμής Ermis). Римляне назвали планету в честь быстроногого римского бога-посланника Меркурия (лат. Mercurius), которого они приравняли к греческому Гермес, потому что он движется по небу быстрее, чем любой другой. планета. астрономический символ Меркурия — это стилизованная версия кадуцея.
Гермеса Греко — египетский астроном Птолемей писал о возможностях планетарных транзитов по лику Солнца в своей работе «Планетарные гипотезы». Эти транзиты были слишком малы, чтобы предположить, что транзиты были слишком малы.
Модель Ибн аль-Шатира для появления Меркурия, показывающая умножение из эпициклов с использованием пары Туси, тем самым устраняя эксцентрики Птолемея и эквант.
В древнем Китае Меркурий известен как «Часовая звезда» «(Чэнь-син 辰星). Это было связано с правлением северной и фазой воды в системе метафизики Пяти фаз. китайские, корейские, японские и вьетнамские культуры называют планету «буквально водной звездой» (水星), сдержанностью на Пять элементов. В индуистской мифологии для Меркурия использовалось имя Будха, считалось, что этот бог правил средой. Бог Один (или Воден) германского язычества был связан с планетой Меркурий и Средой. Майя, возможно, представляла Меркурия в виде совы (или, возможно, четыре сов; две для утреннего аспекта и две вечернего), которая служила посланником в подземный мир.
в средневеко вая исламская астрономия, андалузский астроном Абу Исхак Ибрахим аз-Заркали в XI веке описал отклонение геоцентрической орбиты Меркурия как овальное, как яйцо или пиньон, хотя это понимание не повлияло на его астрономическую теорию или его астрономические расчеты. В XII веке Ибн Баджах наблюдал «две планеты в виде черных пятен на лице Солнца», что позже было предложено как прохождение Меркурия и / или Венеры по Марага астроном Котб ад-Дин Ширази в 13 веке. (Обратите внимание, что большинство средневековых отчетов о транзитах позже были приняты за наблюдения солнечные.)
В Индии Керальская школа астроном Нилаканта Сомаяджи в 15 веке разработал частично гелиоцентрическую модель планеты, в которой Меркурий вращается вокруг Солнца, в свою очередь, вращается вокруг Земли, аналогично системе Тихона, позже предложенной Тихо Браге в конце 16 -го века. века.
Наземные телескопические исследования
Транзит Меркурия. Меркурий виден как черная точка внизу и слева от центра. Темная область над центром солнечного диска — это солнечное пятно.
Удлинение — это угол между Солнцем и планетой с Землей в качестве ориентира. Меркурий кажется близко к Солнцу.
Первые телескопические наблюдения Меркурия были сделаны Галилеем в начале 17 века. Хотя он наблюдал фаза, когда смотрел на Венеру, его телескоп не был достаточно мощным, чтобы увидеть фазы Меркурия. В 1631 году Пьер Гассенди провел первые телескопические наблюдения прохождения планеты через Солнце, когда он увидел прохождение Меркурия, предсказанное Иоганном Кеплером. В 1639 году Джованни Зупи с помощью телескопа обнаружил, что планета имеет орбитальные фазы, подобных Венере и Луне. Наблюдение убежало, что Меркурий вращался вокруг Солнца.
Редким событием в астрономии является проход одной планеты впереди другой (затмение ), если смотреть с Земли. Меркурий и Венера окутывают друг друга через несколько столетий, и событие 28 мая 1737 года — единственное историческое наблюдаемое событие, которое видел Джон Бевис в Гринвичской обсерватории. Следующее покрытие Меркурия Венерой происходит 3 декабря 2133 года.
Трудности, связанные с наблюдением Меркурия, означают, что он намного меньше, чем другие планеты. В 1800 году Иоганн Шретер проводил наблюдения за особенностями поверхности, утверждая, что наблюдал горы высотой 20 километров (12 миль). Фридрих Бессель использовал чертежи Шретера, чтобы ошибочно оценить период вращения как 24 часа и осевой наклон 70 °. В 1880-х годах Джованни Скиапарелли более точно нанес на карту планету и предположил, что период вращения Меркурия составляет 88 дней, что совпадает с периодом его обращения из-за приливной блокировки. Это известно явление как синхронное вращение. Попытки нанести на карту поверхности Меркурия были продолжены Эухениосом Антониади, опубликовавшим в 1934 году книгу, в которую вошли как карты, так и его собственные наблюдения. Многие поверхности планеты, в частности элементы альбедо, получили свои названия из карты Антониади.
В июне 1962 года советские ученые из Института радиотехники и электроники из АН СССР под руководством Владимира Котельникова стали первым, кто отразил сигнал радара от Меркурия и принял его, начав радиолокационные наблюдения планета. Три года спустя радарные наблюдения американцев Гордона Х. Петтенгилла и Рольфа Б. Дайса с использованием 300-метровой обсерватории Аресибо радиотелескопа в Пуэрто Рико убедительно показал, что период вращения планеты составлял около 59 дней. Теория о вращении Меркурия было синхронным, получила широкое распространение, и когда были объявлены эти радионаблюдения, для астрономов стало сюрпризом. Он был заблокирован приливом, но измерения радиоизлучения показали, что он был холодным, чем ожидалось. Астрономы не хотели отказываться от синхронного вращения и предложили альтернативные механизмы, такие как мощные ветры, распространяющие тепло, для объяснения наблюдений.
Водяной лед (желтый) в северной полярной области Меркурия
Итальянский астроном Джузеппе Коломбо отмечает, что величина вращения составляющая около двух третей орбитального периода Меркурия, и предположил, что периоды орбиты и вращения планеты были зафиксированы в резонансе 3: 2, а не 1: 1. Данные с Маринер 10 подтвердили эту точку зрения. Это означает, что карты Скиапарелли и Антониади не были «неправильными». Вместо этого астрономы видели одни и те же детали на каждой второй орбите и записывали их, но игнорировали те, которые наблюдались тем временем, когда другая сторона обращалась к Солнцу, потому что геометрия орбиты означала, что эти наблюдения проводились в условиях плохой видимости.
Наземные оптические наблюдения не пролили много света на Меркурий, но радиоастрономы, используя их интерферометрию на микроволновых волн, метод, позволяющий удалить солнечное излучение, смогли различить физические и химические характеристики подповерхностных слоев, чтобы определить. глубина в несколько метров. Только после того, как первый космический зонд пролетел мимо Меркурия, стали известны многие из его фундаментальных морфологических свойств. Более того, последние технологические достижения привели к улучшению наземных наблюдений. В 2000 году с помощью 1,5-метрового телескопа Хейла обсерватории Маунт-Вилсон были проведены наблюдения с высоким разрешением удачными изображениями. Они предоставили первые изображения, которые позволили разрешить особенности поверхности частей Меркурия, которые не были отображены в миссии Mariner 10. Большая часть планеты была нанесена на карту с помощью радиолокационного телескопа Аресибо с разрешением 5 км (3,1 мили), включая полярные отложения в затененных кратерах того, что может быть водяным льдом.
Исследования с помощью космических зондов
MESSENGER готовится к запуску
Меркурий, проходящий мимо Солнца, с точки зрения марсохода Марс Curiosity (3 июня 2014 г.)
Достижение Меркурия с Земли связано со значительными техническими проблемами, потому что он вращается намного ближе к Солнцу, чем Земля. Связанный с Меркурием космический корабль, запущенный с Земли, должен пройти более 91 миллиона километров (57 миллионов миль) в гравитационную потенциальную яму Солнца. Орбитальная скорость Меркурия составляет 48 км / с (30 миль / с), тогда как орбитальная скорость Земли составляет 30 км / с (19 миль / с). Следовательно, космический корабль должен сильно изменить скорость (delta-v ), чтобы добраться до Меркурия и затем выйти на орбиту, по сравнению с delta-v, требуемым для других планетарных миссий.
потенциальная энергия, высвобождаемая при движении вниз по потенциальной яме Солнца, становится кинетической энергией ; требуется еще одно большое изменение дельта-v для чего-либо, кроме быстрого прохождения мимо Меркурия. Чтобы безопасно приземлиться или выйти на стабильную орбиту, космический корабль будет полностью полагаться на ракетные двигатели. Аэротормоз исключен, поскольку у Меркурия незначительная атмосфера. Для полета к Меркурию требуется больше ракетного топлива, чем требуется для полного выхода из Солнечной системы. В результате его пока посетили всего два космических зонда. Предлагаемый альтернативный подход предполагает использование солнечного паруса для выхода на синхронную с Меркурием орбиту вокруг Солнца.
Mariner 10
Mariner 10, первый зонд, посетивший Меркурий
Первый космический корабль, который Визит Меркурий был НАСА Маринер-10 (1974–1975). Космический корабль использовал гравитацию Венеры, чтобы отрегулировать свою орбитальную скорость так, чтобы он мог приблизиться к Меркурию, что сделало его первым космическим кораблем, использующим этот эффект гравитационной «рогатки», и первой миссией НАСА. посетить несколько планет. Mariner 10 предоставил первые изображения поверхности Меркурия крупным планом, которые сразу показали его сильно изрезанную кратерами природу и выявили многие другие типы геологических особенностей, такие как гигантские уступы, которые позже были приписаны эффекту небольшой усадки планеты, как ее железа. ядро остывает. К сожалению, одно и то же лицо планеты освещалось при каждом приближении Mariner 10. Это сделало невозможным тщательное наблюдение за обеими сторонами планеты, и в результате было нанесено на карту менее 45% поверхности планеты.
Космический корабль сделал три сближения с Меркурием, самый близкий из которых доставил его внутрь 327 км (203 мили) поверхности. При первом приближении приборы обнаружили магнитное поле, к большому удивлению планетных геологов: ожидалось, что вращение Меркурия будет слишком медленным, чтобы вызвать значительный эффект динамо. Второй подход в основном использовался для получения изображений, но с помощью третьего подхода были получены обширные магнитные данные. Данные показали, что магнитное поле планеты очень похоже на магнитное поле Земли, которое отклоняет солнечный ветер вокруг планеты. В течение многих лет после столкновений с Mariner 10 происхождение магнитного поля Меркурия оставалось предметом нескольких конкурирующих теорий.
24 марта 1975 года, всего через восемь дней после последнего сближения, у Mariner 10 закончилось топливо.. Поскольку его орбиту больше нельзя было точно контролировать, диспетчеры миссии приказали зонду выключиться. Считается, что Mariner 10 все еще вращается вокруг Солнца, проходя мимо Меркурия каждые несколько месяцев.
MESSENGER
Предполагаемые детали воздействия MESSENGER 30 апреля 2015 г.
Вторая миссия НАСА к Меркурию, названный MESSENGER (Меркурийная поверхность, космическая среда, геохимия и ранжирование), был запущен 3 августа 2004 г. Он пролетел мимо Земли в августе 2005 г. и Венеры в октябре 2006 г. и июне 2007 г., чтобы поместить его на правильная траектория для выхода на орбиту вокруг Меркурия. Первый пролет Меркурия произошел 14 января 2008 г., второй — 6 октября 2008 г., а третий — 29 сентября 2009 г. Большая часть полушария, не отображаемая Mariner 10, была нанесена на карту во время этих пролетов. Зонд успешно вышел на эллиптическую орбиту вокруг планеты 18 марта 2011 г. Первое орбитальное изображение Меркурия было получено 29 марта 2011 г. Зонд завершил однолетнюю миссию по составлению карт, а затем приступил к однолетней расширенной миссии в 2013. В дополнение к постоянным наблюдениям и картированию Меркурия, MESSENGER наблюдал в 2012 году солнечный максимум.
. Миссия была разработана, чтобы прояснить шесть ключевых вопросов: высокая плотность Меркурия, его геологическая история, природа его магнитное поле, структура его ядра, наличие льда на полюсах и источник его разреженной атмосферы. С этой целью на зонд были установлены устройства для получения изображений, которые собирали изображения с гораздо большим разрешением и гораздо большего количества Меркурия, чем у Mariner 10, различные спектрометры для определения содержания элементов в коре и магнитометры и устройства для измерения скорости заряженных частиц. Ожидалось, что измерения изменений в орбитальной скорости зонда будут использоваться для определения деталей внутренней структуры планеты. Последний маневр MESSENGER состоялся 24 апреля 2015 года, и он врезался в поверхность Меркурия 30 апреля 2015 года. Столкновение космического корабля с Меркурием произошло около 15:26 по восточному поясному времени 30 апреля 2015 года, в результате чего образовался кратер высотой 16 м (52 футов) в диаметре.
Первое (29 марта 2011 г.) и последнее (30 апреля 2015 г.) изображения Меркурия, сделанные MESSENGER
BepiColombo
Европейским космическим агентством и Японское космическое агентство разработало и запустило совместную миссию под названием BepiColombo, которая будет вращаться вокруг Меркурия с двумя зондами: один для составления карты планеты, а другой для изучения ее магнитосферы. Запущенный 20 октября 2018 года, BepiColombo, как ожидается,достигнет Меркурия в 2025 году. Он выведет зонд с магнитометром на эллиптическую орбиту, а затем запустят химические ракеты, выведя зонд-картограф на круговую орбиту. Оба зонда будут работать в течение одного земного года. Зонд-картограф оснащен набором спектрометров, аналогичных спектрометрам на MESSENGER, и будет изучать планету на многих различных длинах волн, включая инфракрасный, ультрафиолетовый, рентгеновский и гамма-луч.
Сравнение
Сравнение размеров с другими объектами Солнечной системы Меркурий, Земля
Меркурий, Венера, Земля, Марс
Задний ряд: Марс, Меркурий. Передний: Луна, Плутон, Хаумеа
См. Также
Портал Солнечной системы
- Схема Меркурия (планета)
- Будха, индуистское название планеты и бога Меркурия
- Колонизация Меркурия
- Исследование Меркурия
- Меркурий в астрологии
- Меркурий в художественной литературе
- Хронология далекого будущего
- Холодная ловушка (астрономия)
Примечания
Ссылки
Внешние ссылки
Слушайте эту статью Этот аудиофайл был создан на основе редакции этой статьи от 16 января 2008 г. и не смотрит правок. (
- Аудиопомощь
- Другие устные статьи
)
- Atl по состоянию на Меркурий. НАСА. 1978. SP-423.
- Номенклатура Меркурия и карта с названиями объектов из Справочника по планетарной номенклатуре USGS / IAU
- Равнопрямоугольная карта Меркурия от Applied Coherent Technology Corp
- 3D-глобус Меркурия от Google
- Меркурия на Solarviews.com
- Меркурий от Astronomy Cast
- Веб-сайт миссии MESSENGER
- Веб-сайт миссии BepiColombo