Какой химический элемент составляет основу алмаза-вопрос,интересующий многих.И ответ очень простой-это C, углерод,химический элемент,имеющий в таблице Менделеева шестой порядковый номер.Количество примесей в алмазе настолько мало,что они не учитываются в его химической формуле.Надо отметить,что алмаз состоит из атомов,а не из молекул углерода.Итак,химическая формула алмаза-C.
Если говорить о примесях более подробно,то чаще всего встречаются азот,кремний,магний,кальций,аллюминий,бор.Реже-железо,барий,медь и другие.В процентном выражении максимальное количество примесей может составлять 5%.Один компонент примесей не более 2%.
Уникальность алмаза объясняется строением его кристаллической решётки.Алмаз кристаллизуется в кубической системе,отвечающей самой плотной «упаковке» атомов,и содержащей всего 18 атомов углерода.В ней каждый атом связан с четырьмя ближайшими атомами,расположенными в вершинах правильного тетраэдра.Расстояние между двумя соседними атомами решётки 0,154 нм.Постоянный размер кристаллической решётки 0,356 нм.
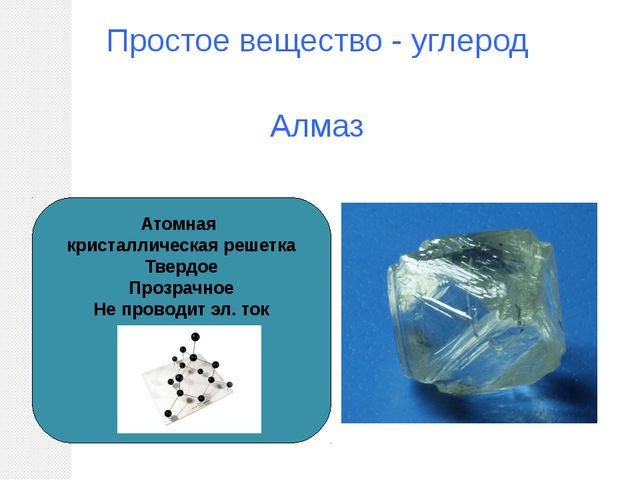
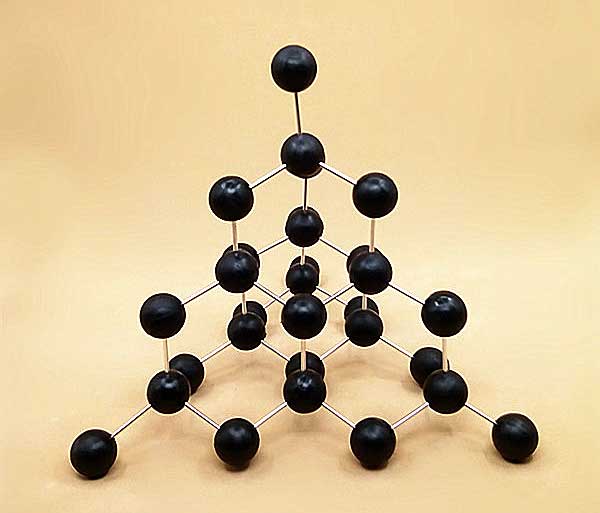
От валентного состояния, в котором находятся атомы углерода в веществе, зависят свойства этого вещества. Поэтому углерод может принимать разные,аллотропные, формы:сажа,древесный уголь,графит,графен,кокс,алмаз гексагональный,углеродные нанотрубки,поликумулен,фуллерен.
Разница между ними в строении кристаллической решетки.Например, кристаллическая решетка графита — слоистого типа. В слоях атомы С расположены в узлах гексагональных ячеек слоя. Каждый атом С окружен тремя соседними с расстоянием 1,42Α
В чем уникальность алмаза?
-Алмаз не имеет аналогов ни природных,ни искусственно созданных.Он имеет наивысшую твёрдость среди минералов по десятибалльной шкале твёрдости Мооса и имеет значение 10 из 10.
-В силу своей твёрдости алмаз очень инертен,имеет очень высокую устойчивость к воздействию кислот и щелочей.
-Алмаз имеет очень высокую теплопроводность.Не нагревается в руках.
-Также можно отметить такие свойства алмаза,как
отсутствие электропроводности, низкая фотопроводимость, аномальное двупреломление.
-Но при этом алмаз хрупкий,его можно разбить
-Горит в присутствии кислорода при температуре 750-800 °
-Под воздействием рентгеновского облучения сам начинает излучать свет зеленого или синего оттенка
-Отталкивает воду,но прилипает к жиру
-Имеет высокую износостойкость
Происхождение алмазов.
В основном считается,что алмазы образовались в недрах планеты при воздействии высоких температур и давлении.Хотя есть теория о их внеземном происхождении,попадании на землю при массовой бомбардировке метеоритами.
Алмазы образовались в так называемых кимберлитовых трубках (от фамилии Кимберли) миллионы,миллиарды лет назад.Потом под действием эрозии часть из них попала в реки и была разнесена вдоль их русел.Там и были найдены людьми первые алмазы.В истории об алмазах заговорили более 5 тысяч лет назад,когда жители Индии обратили внимание на блестящие камни, которые невозможно было разбить, но с их помощью легко обрабатывались другие минералы.
Природный алмаз привлекал древних жителей Индии своей прозрачностью и блеском, постепенно став своеобразными твердыми деньгами, за которые можно было приобретать еду, одежду и другие ценности. Правители страны не подвергали эти камни никакой обработке, а хранили в целом виде, помещая в сокровищницы. В Европе о существовании кристаллов алмаза узнали лишь в эпоху Александра Македонского после его походов в Индию.И нынешнее название алмаз произошло от греческого «Адамас»,от которого произошло арабское «Алмас» и затем уже привычное «Алмаз».
И в наше время часть алмазов находится в рассыпных месторождениях,но главным образом алмазы добываются шахтным способом.
Алмазы можно получать также искусственным способом.Существует несколько путей получения.
-создание условий,близких к природным,так называемая HPHT технология.Алмаз синтезируется из графита,оседающего на затравочном камне
-химическое парофазное осаждение,или CVD метод,получение алмаза из паров метана.При этом способе также происходит осаждение графита.
-взрывной синтез.Переход графита в алмаз при при ударных нагрузках
Все эти способы не дают возможности получить алмазы,близкие по качеству к натуральным,поэтому они используются для технических целей.
Тем не менее,в последнее время удалось добиться значительного прогресса в производстве муассанита,одного из видов искусственного алмаза.Муассанит превосходит по своим свойствам настоящий алмаз по искристости,так как коэффициент преломления света у него на 25% выше,чем у природного камня.Сейчас уже возможно получение полностью прозрачного муассанита,и в ювелирном деле он просто затмил природный алмаз.
Алмазы широко применяются в ювелирном деле и в промышленности.
Содержание
- Формула и структура минерала
- Характеристики вещества
- Химические и физические свойства
- Особенности внешнего вида
- Добыча и производство
- В природных условиях
- Искусственный синтез
- Применение алмаза
Алмаз — это простое вещество-неметалл, которое почти полностью состоит из углерода. Этот минерал известен благодаря своему широкому использованию в ювелирном деле, а также из-за своих необычных физических свойств, среди которых выделяется высочайшая прочность. При этом химическая формула алмаза такая же, как у обычного графита — C, а его уникальность и ценность обусловлена исключительно строением кристаллической решётки.
Формула и структура минерала
Углерод, он же карбон — это элемент, имеющий в таблице Менделеева шестой порядковый номер и записывающийся как C. Именно из него целиком состоит алмаз — количество примесей, если они есть, настолько мало, что они не учитываются в формуле. Помимо алмаза, углерод принимает такие аллотропные, то есть состоящие из одного вещества, формы:
- древесный уголь;
- сажа;
- графит;
- графен;
- карбин;
- кокс;
- алмаз гексагональный, или лонсдейлит;
- фуллерен;
- поликумулен;
- УНТ или углеродные нанотрубки.
Некоторым трудно поверить, что прозрачный и прочный алмаз имеет одинаковый состав с обычной сажей, углём или графитом, но это так. Дело в том, что, в отличие от прочих веществ, состоящих из углерода, атомы алмаза образуют кубическую упорядоченную структуру, чем и объясняются его необычные качества и внешний вид.
Кристаллическая решётка минерала имеет кубическую форму. Каждый атом в её структуре находится в центре тетраэдра, вершины которого представляют собой 4 других атома, при этом между ними образуется надёжная сигма-связь. Расстояние между всеми атомами одинаково и составляет примерно 0,15 нм. Кроме того, для решётки минерала характерна кубическая симметрия. Все эти качества и особенности структурной формулы алмаза и обусловливают его огромную прочность по сравнению с веществами-«родственниками».
Характеристики вещества
Алмаз — это очень необычное вещество, не имеющее ни природных, ни искусственно созданных аналогов. Его происхождение неизвестно, но есть несколько гипотез, среди которых выделяются две наиболее правдоподобные: нагревание карбона при высоком давлении из-за подъёма магмы на поверхность планеты и падение на Землю большого количества содержащих этот минерал метеоритов.
От подделок и имитаций настоящий драгоценный камень отличают с помощью «алмазного щупа», который измеряет его теплопроводность. Ещё иногда используют пробу специальным жировым фломастером, который должен оставлять на поверхности минерала сплошную линию. Оба метода позволяют подтвердить наличие у вещества особенностей, характерных только для подлинного алмаза.
Химические и физические свойства
Стоит отметить, что алмаз состоит из атомов, а не из молекул углерода — последние очень редки и в природе почти не встречаются. Кроме того, строение драгоценного камня наделило его множеством уникальных свойств, среди которых выделяются:
Наивысшая твёрдость среди минералов по шкале Мооса — 3,47 — 3,55 г/см³. Это значит, что поцарапать поверхность алмаза способен только другой алмаз.
- При этом минерал очень хрупок, и его можно разбить. Раскалывается кристалл строго по определённым плоскостям.
- Ещё для него характерна очень высокая устойчивость к воздействию как кислотной, так и щелочной среды, а также отличная износостойкость.
- Минерал отталкивает воду, но при этом прилипает к жиру.
- При обычном давлении алмаз не плавится, а сублимирует (сразу превращается в газ, минуя жидкое состояние) при температуре в примерно 3500 °C. Если нагревание проводится при отсутствии кислорода с давлением в 11 ГПа, его температура плавления составляет 3700—4000 °C.
- При этом если есть свободный доступ кислорода, минерал горит при температуре всего 750—800 °C. В процессе сгорания он выделяет углекислоту.
- Ещё для него характерна очень высокая теплопроводность. Благодаря ей минерал не нагревается в руках — это самый простой способ проверить его подлинность. Кроме того, у вещества плохая проводимость электричества.
- Отличает алмаз и крайне небольшой коэффициент трения по металлу: если присутствует воздух, оно составляет всего 0,1. Ещё у него самая низкая среди всех веществ способность к деформации (и, соответственно, самый высокий модуль упругости) и сжимаемость.
Особенности внешнего вида
Алмаз известен в первую очередь благодаря своему внешнему виду, сделавшему его очень популярным и роскошным ювелирным украшением. Среди прочих драгоценных минералов его выделяют высокие показатели прозрачности и преломления цвета, а также дисперсии. Именно благодаря ей огранённый камень обладает очень красивой люминесценцией, то есть сверкает всеми цветами радуги, переливаясь на свету.
В идеале драгоценный камень должен состоять из чистого углерода, но в природе такое встречается редко. Примеси в минерале могут как отрицательно влиять на его качество, снижая цену, так и придавать ему красивые и необычные оттенки. Ещё на цвет иногда может повлиять радиоактивное излучение. Обычно натуральные алмазы — жёлтые, но встречаются и голубые, синие, зелёные, розовые и даже красные экземпляры. Несмотря на разнообразие оттенков, чистый минерал всегда должен быть прозрачен.
В природе алмазы имеют самые разные формы. Чаще всего встречается кристалл с восемью гранями в форме правильного треугольника. На втором месте — ромбододекаэдр с двенадцатью гранями. Бывают также кубические и круглые камни, но они очень редки. При огранке минерала потери вещества стараются свести к минимуму, чтобы максимизировать прибыль от производства бриллиантов.
Добыча и производство
История добычи и продажи этих драгоценных камней насчитывает тысячи лет. Впервые их обнаружили в Индии — точная дата неизвестна, но описание кристалла было сделано ещё в IV до н. э. Ещё тогда он считался очень ценным, даже несмотря на то, что люди того времени не умели гранить минерал и только шлифовали его. Известно, что алмазы были популярны ещё и в древнем Египте, а в Европу их завезли во времена Александра Македонского.
Гранить кристаллы научились с XVII века, и с тех пор это искусство постоянно совершенствовалось. На протяжении столетий появлялись всё новые формы и способы обработки драгоценного минерала, до тех пор, пока в 1919 году Марсель Толковский не изобрёл идеальную огранку бриллиантов, которую применяют и сейчас.
В природных условиях
Алмазы относятся к природным ископаемым. Основная часть драгоценных камней — около 90% — содержится в кимберлитовых трубках, также их некоторое количество можно найти в лампроитах и россыпях. Наличие этого ценного минерала в горной породе определяют с помощью рентгеновских лучей. При этом бо́льшая часть добытых камней идёт не в ювелирное дело, а на промышленность — это происходит из-за наличия в них большого количества (>5% в целом или >2% вещества одного типа) примесей, а также структурных дефектов. Промышленные кристаллы бывают таких типов:
- борт — мелкая алмазная крошка, которую невозможно огранить, используется для резки некоторых огнеупорных материалов;
- баллас — мелкозернистые кристаллы, оболочка которых твёрже ядра, обычно имеют мутно-белый или серый цвет и используются для производства буровых коронок;
- карбонадо — непрозрачный или полупрозрачный чёрный алмаз, похожий на уголь, обычно содержит много примесей железа, графита или сульфитов.
Искусственный синтез
В лабораториях можно получить алмаз из графита. Это достигает путём влияния на его кристаллическую решётку, но такой переход представляет собой очень дорогой, трудоёмкий и энергозатратный процесс. Более дешёвые кристаллы, по своим свойствам напоминающие алмаз, можно искусственно выращивать. В целом лабораторное создание этих драгоценных камней можно поделить на три типа:
- HPTH (High Temperature High Pressure, что переводится как высокая температура и давление) — создание условий, похожих на природную среду формирования минерала, с помощью особых приборов. Синтез вещества при этом происходит из графита, оседающего на кристаллической решётке затравочного алмаза.
- CVD (Chemical vapor deposition или химическое парофазное осаждение) — получение вещества из паров метана химическим путём. Этот способ также использует осаждения графита.
- Взрывной синтез — переход структуры углерода из графита в алмаз при ударно-волновом нагружении.
Ни один из этих способов пока что не может создать камни, по своему качеству близкие к натуральным, потому их редко используют в ювелирном деле. Кроме того, все виды производства далеки от массовых, потому старания учёных в этом деле пока продолжаются.
Применение алмаза
Огранённый алмаз, он же бриллиант — это очень популярный драгоценный камень, являющийся также одним из самых дорогих. Хотя на продажу в качестве ювелирных украшений поступает только малая часть минерала, именно она составляет основную часть дохода с его добычи. В значительной степени дороговизна камня обусловлена монополизацией производства бриллиантов — более 50% их оборота принадлежит одной компании.
Помимо ювелирного дела, есть ещё множество отраслей, в которой минерал нашёл своё применение. В основном ценится его высочайшая прочность, обусловленная структурной формулой алмаза. В химии его применяют для защиты от кислот и некоторых очень едких веществ. В промышленности алмазную плёнку наносят на резцы, свёрла, ножи, части добывающих установок и другие подобные инструменты Алмазную крошку используют как абразив для шлифования точильных поверхностей.
Используют его и в создании медицинских инструментов, так как острота и прочность алмазных изделий может обеспечить максимально точные надрезы при операциях. Ещё его применяют для изготовления деталей для современных квантовых компьютеров и часов, в ядерной промышленности, а также во многих других отраслях.
Diamond | |
---|---|
![]() The slightly misshapen octahedral shape of this rough diamond crystal in matrix is typical of the mineral. Its lustrous faces also indicate that this crystal is from a primary deposit. | |
General | |
Category | Native minerals |
Formula (repeating unit) | C |
IMA symbol | Dia[1] |
Strunz classification | 1.CB.10a |
Dana classification | 1.3.6.1 |
Crystal system | Cubic |
Crystal class | Hexoctahedral (m3m) H-M symbol: (4/m 3 2/m) |
Space group | Fd3m (No. 227) |
Structure | |
Jmol (3D) | Interactive image |
Identification | |
Formula mass | 12.01 g/mol |
Color | Typically yellow, brown, or gray to colorless. Less often blue, green, black, translucent white, pink, violet, orange, purple, and red. |
Crystal habit | Octahedral |
Twinning | Spinel law common (yielding «macle») |
Cleavage | 111 (perfect in four directions) |
Fracture | Irregular/Uneven |
Mohs scale hardness | 10 (defining mineral) |
Luster | Adamantine |
Streak | Colorless |
Diaphaneity | Transparent to subtransparent to translucent |
Specific gravity | 3.52±0.01 |
Density | 3.5–3.53 g/cm3 3500–3530 kg/m3 |
Polish luster | Adamantine |
Optical properties | Isotropic |
Refractive index | 2.418 (at 500 nm) |
Birefringence | None |
Pleochroism | None |
Dispersion | 0.044 |
Melting point | Pressure dependent |
References | [2][3] |
Main diamond producing countries
Diamond is a solid form of the element carbon with its atoms arranged in a crystal structure called diamond cubic. Another solid form of carbon known as graphite is the chemically stable form of carbon at room temperature and pressure, but diamond is metastable and converts to it at a negligible rate under those conditions. Diamond has the highest hardness and thermal conductivity of any natural material, properties that are used in major industrial applications such as cutting and polishing tools. They are also the reason that diamond anvil cells can subject materials to pressures found deep in the Earth.
Because the arrangement of atoms in diamond is extremely rigid, few types of impurity can contaminate it (two exceptions are boron and nitrogen). Small numbers of defects or impurities (about one per million of lattice atoms) color diamond blue (boron), yellow (nitrogen), brown (defects), green (radiation exposure), purple, pink, orange, or red. Diamond also has a very high refractive index and a relatively high optical dispersion.
Most natural diamonds have ages between 1 billion and 3.5 billion years. Most were formed at depths between 150 and 250 kilometres (93 and 155 mi) in the Earth’s mantle, although a few have come from as deep as 800 kilometres (500 mi). Under high pressure and temperature, carbon-containing fluids dissolved various minerals and replaced them with diamonds. Much more recently (hundreds to tens of million years ago), they were carried to the surface in volcanic eruptions and deposited in igneous rocks known as kimberlites and lamproites.
Synthetic diamonds can be grown from high-purity carbon under high pressures and temperatures or from hydrocarbon gases by chemical vapor deposition (CVD). Imitation diamonds can also be made out of materials such as cubic zirconia and silicon carbide. Natural, synthetic and imitation diamonds are most commonly distinguished using optical techniques or thermal conductivity measurements.
Properties
Diamond is a solid form of pure carbon with its atoms arranged in a crystal. Solid carbon comes in different forms known as allotropes depending on the type of chemical bond. The two most common allotropes of pure carbon are diamond and graphite. In graphite the bonds are sp2 orbital hybrids and the atoms form in planes, with each bound to three nearest neighbors 120 degrees apart. In diamond they are sp3 and the atoms form tetrahedra with each bound to four nearest neighbors.[4][5] Tetrahedra are rigid, the bonds are strong, and of all known substances diamond has the greatest number of atoms per unit volume, which is why it is both the hardest and the least compressible.[6][7] It also has a high density, ranging from 3150 to 3530 kilograms per cubic metre (over three times the density of water) in natural diamonds and 3520 kg/m3 in pure diamond.[2] In graphite, the bonds between nearest neighbors are even stronger, but the bonds between parallel adjacent planes are weak, so the planes easily slip past each other. Thus, graphite is much softer than diamond. However, the stronger bonds make graphite less flammable.[8]
Diamonds have been adopted for many uses because of the material’s exceptional physical characteristics. It has the highest thermal conductivity and the highest sound velocity. It has low adhesion and friction, and its coefficient of thermal expansion is extremely low. Its optical transparency extends from the far infrared to the deep ultraviolet and it has high optical dispersion. It also has high electrical resistance. It is chemically inert, not reacting with most corrosive substances, and has excellent biological compatibility.[9]
Thermodynamics
The equilibrium pressure and temperature conditions for a transition between graphite and diamond are well established theoretically and experimentally. The equilibrium pressure varies linearly with temperature, between 1.7 GPa at 0 K and 12 GPa at 5000 K (the diamond/graphite/liquid triple point).[10][11]
However, the phases have a wide region about this line where they can coexist. At normal temperature and pressure, 20 °C (293 K) and 1 standard atmosphere (0.10 MPa), the stable phase of carbon is graphite, but diamond is metastable and its rate of conversion to graphite is negligible.[7] However, at temperatures above about 4500 K, diamond rapidly converts to graphite. Rapid conversion of graphite to diamond requires pressures well above the equilibrium line: at 2000 K, a pressure of 35 GPa is needed.[10]
Above the graphite-diamond-liquid carbon triple point, the melting point of diamond increases slowly with increasing pressure; but at pressures of hundreds of GPa, it decreases.[12] At high pressures, silicon and germanium have a BC8 body-centered cubic crystal structure, and a similar structure is predicted for carbon at high pressures. At 0 K, the transition is predicted to occur at 1100 GPa.[13]
Research results published in an article in the scientific journal Nature Physics in 2010 suggest that at ultrahigh pressures and temperatures (about 10 million atmospheres or 1 TPa and 50,000 °C) diamond melts into a metallic fluid. The extreme conditions required for this to occur are present in the ice giants Neptune and Uranus. Both planets are made up of approximately 10 percent carbon and could hypothetically contain oceans of liquid carbon. Since large quantities of metallic fluid can affect the magnetic field, this could serve as an explanation as to why the geographic and magnetic poles of the two planets are unaligned.[14][15]
Crystal structure
Diamond unit cell, showing the tetrahedral structure
The most common crystal structure of diamond is called diamond cubic. It is formed of unit cells (see the figure) stacked together. Although there are 18 atoms in the figure, each corner atom is shared by eight unit cells and each atom in the center of a face is shared by two, so there are a total of eight atoms per unit cell.[16] The length of each side of the unit cell is denoted by a and is 3.567 angstroms.[17]
The nearest neighbour distance in the diamond lattice is 1.732a/4 where a is the lattice constant, usually given in Angstrøms as a = 3.567 Å, which is 0.3567 nm.
A diamond cubic lattice can be thought of as two interpenetrating face-centered cubic lattices with one displaced by 1⁄4 of the diagonal along a cubic cell, or as one lattice with two atoms associated with each lattice point.[17] Viewed from a <1 1 1> crystallographic direction, it is formed of layers stacked in a repeating ABCABC … pattern. Diamonds can also form an ABAB … structure, which is known as hexagonal diamond or lonsdaleite, but this is far less common and is formed under different conditions from cubic carbon.[18]
Crystal habit
One face of an uncut octahedral diamond, showing trigons (of positive and negative relief) formed by natural chemical etching
Diamonds occur most often as euhedral or rounded octahedra and twinned octahedra known as macles. As diamond’s crystal structure has a cubic arrangement of the atoms, they have many facets that belong to a cube, octahedron, rhombicosidodecahedron, tetrakis hexahedron, or disdyakis dodecahedron. The crystals can have rounded-off and unexpressive edges and can be elongated. Diamonds (especially those with rounded crystal faces) are commonly found coated in nyf, an opaque gum-like skin.[19]
Some diamonds contain opaque fibers. They are referred to as opaque if the fibers grow from a clear substrate or fibrous if they occupy the entire crystal. Their colors range from yellow to green or gray, sometimes with cloud-like white to gray impurities. Their most common shape is cuboidal, but they can also form octahedra, dodecahedra, macles, or combined shapes. The structure is the result of numerous impurities with sizes between 1 and 5 microns. These diamonds probably formed in kimberlite magma and sampled the volatiles.[20]
Diamonds can also form polycrystalline aggregates. There have been attempts to classify them into groups with names such as boart, ballas, stewartite, and framesite, but there is no widely accepted set of criteria.[20] Carbonado, a type in which the diamond grains were sintered (fused without melting by the application of heat and pressure), is black in color and tougher than single crystal diamond.[21] It has never been observed in a volcanic rock. There are many theories for its origin, including formation in a star, but no consensus.[20][22][23]
Mechanical
Hardness
The extreme hardness of diamond in certain orientations makes it useful in materials science, as in this pyramidal diamond embedded in the working surface of a Vickers hardness tester.
Diamond is the hardest known natural material on both the Vickers scale and the Mohs scale. Diamond’s great hardness relative to other materials has been known since antiquity, and is the source of its name. This does not mean that it is infinitely hard, indestructible, or unscratchable.[24] Indeed, diamonds can be scratched by other diamonds[25] and worn down over time even by softer materials, such as vinyl phonograph records.[26]
Diamond hardness depends on its purity, crystalline perfection, and orientation: hardness is higher for flawless, pure crystals oriented to the <111> direction (along the longest diagonal of the cubic diamond lattice).[27] Therefore, whereas it might be possible to scratch some diamonds with other materials, such as boron nitride, the hardest diamonds can only be scratched by other diamonds and nanocrystalline diamond aggregates.
The hardness of diamond contributes to its suitability as a gemstone. Because it can only be scratched by other diamonds, it maintains its polish extremely well. Unlike many other gems, it is well-suited to daily wear because of its resistance to scratching—perhaps contributing to its popularity as the preferred gem in engagement or wedding rings, which are often worn every day.
The hardest natural diamonds mostly originate from the Copeton and Bingara fields located in the New England area in New South Wales, Australia. These diamonds are generally small, perfect to semiperfect octahedra, and are used to polish other diamonds. Their hardness is associated with the crystal growth form, which is single-stage crystal growth. Most other diamonds show more evidence of multiple growth stages, which produce inclusions, flaws, and defect planes in the crystal lattice, all of which affect their hardness. It is possible to treat regular diamonds under a combination of high pressure and high temperature to produce diamonds that are harder than the diamonds used in hardness gauges.[28]
Diamonds cut glass, but this does not positively identify a diamond because other materials, such as quartz, also lie above glass on the Mohs scale and can also cut it. Diamonds can scratch other diamonds, but this can result in damage to one or both stones. Hardness tests are infrequently used in practical gemology because of their potentially destructive nature.[29] The extreme hardness and high value of diamond means that gems are typically polished slowly, using painstaking traditional techniques and greater attention to detail than is the case with most other gemstones;[30] these tend to result in extremely flat, highly polished facets with exceptionally sharp facet edges. Diamonds also possess an extremely high refractive index and fairly high dispersion. Taken together, these factors affect the overall appearance of a polished diamond and most diamantaires still rely upon skilled use of a loupe (magnifying glass) to identify diamonds «by eye».[31]
Toughness
Somewhat related to hardness is another mechanical property toughness, which is a material’s ability to resist breakage from forceful impact. The toughness of natural diamond has been measured as 7.5–10 MPa·m1/2.[32][33] This value is good compared to other ceramic materials, but poor compared to most engineering materials such as engineering alloys, which typically exhibit toughnesses over 100 MPa·m1/2. As with any material, the macroscopic geometry of a diamond contributes to its resistance to breakage. Diamond has a cleavage plane and is therefore more fragile in some orientations than others. Diamond cutters use this attribute to cleave some stones, prior to faceting.[34] «Impact toughness» is one of the main indexes to measure the quality of synthetic industrial diamonds.
Yield strength
Diamond has compressive yield strength of 130–140 GPa.[35] This exceptionally high value, along with the hardness and transparency of diamond, are the reasons that diamond anvil cells are the main tool for high pressure experiments.[36] These anvils have reached pressures of 600 GPa.[37] Much higher pressures may be possible with nanocrystalline diamonds.[36][37]
Elasticity and tensile strength
Usually, attempting to deform bulk diamond crystal by tension or bending results in brittle fracture. However, when single crystalline diamond is in the form of micro/nanoscale wires or needles (~100–300 nanometers in diameter, micrometers long), they can be elastically stretched by as much as 9-10 percent tensile strain without failure,[38] with a maximum local tensile stress of ~89 to 98 GPa,[39] very close to the theoretical limit for this material.[40]
Electrical conductivity
Other specialized applications also exist or are being developed, including use as semiconductors: some blue diamonds are natural semiconductors, in contrast to most diamonds, which are excellent electrical insulators. The conductivity and blue color originate from boron impurity. Boron substitutes for carbon atoms in the diamond lattice, donating a hole into the valence band.[41]
Substantial conductivity is commonly observed in nominally undoped diamond grown by chemical vapor deposition. This conductivity is associated with hydrogen-related species adsorbed at the surface, and it can be removed by annealing or other surface treatments.[42][43]
Thin needles of diamond can be made to vary their electronic band gap from the normal 5.6 eV to near zero by selective mechanical deformation.[44]
High-purity diamond wafers 5 cm in diameter exhibit perfect resistance in one direction and perfect conductance in the other, creating the possibility of using them for quantum data storage. The material contains only 3 parts per million of nitrogen. The diamond was grown on a stepped substrate, which eliminated cracking.[45]
Surface property
Diamonds are naturally lipophilic and hydrophobic, which means the diamonds’ surface cannot be wet by water, but can be easily wet and stuck by oil. This property can be utilized to extract diamonds using oil when making synthetic diamonds. However, when diamond surfaces are chemically modified with certain ions, they are expected to become so hydrophilic that they can stabilize multiple layers of water ice at human body temperature.[46]
The surface of diamonds is partially oxidized. The oxidized surface can be reduced by heat treatment under hydrogen flow. That is to say, this heat treatment partially removes oxygen-containing functional groups. But diamonds (sp3C) are unstable against high temperature (above about 400 °C (752 °F)) under atmospheric pressure. The structure gradually changes into sp2C above this temperature. Thus, diamonds should be reduced under this temperature.[47]
Chemical stability
At room temperature, diamonds do not react with any chemical reagents including strong acids and bases.
In an atmosphere of pure oxygen, diamond has an ignition point that ranges from 690 °C (1,274 °F) to 840 °C (1,540 °F); smaller crystals tend to burn more easily. It increases in temperature from red to white heat and burns with a pale blue flame, and continues to burn after the source of heat is removed. By contrast, in air the combustion will cease as soon as the heat is removed because the oxygen is diluted with nitrogen. A clear, flawless, transparent diamond is completely converted to carbon dioxide; any impurities will be left as ash.[48] Heat generated from cutting a diamond will not ignite the diamond,[49] and neither will a cigarette lighter,[50] but house fires and blow torches are hot enough. Jewelers must be careful when molding the metal in a diamond ring.[51]
Diamond powder of an appropriate grain size (around 50 microns) burns with a shower of sparks after ignition from a flame. Consequently, pyrotechnic compositions based on synthetic diamond powder can be prepared. The resulting sparks are of the usual red-orange color, comparable to charcoal, but show a very linear trajectory which is explained by their high density.[52] Diamond also reacts with fluorine gas above about 700 °C (1,292 °F).
Color
Diamond has a wide band gap of 5.5 eV corresponding to the deep ultraviolet wavelength of 225 nanometers. This means that pure diamond should transmit visible light and appear as a clear colorless crystal. Colors in diamond originate from lattice defects and impurities. The diamond crystal lattice is exceptionally strong, and only atoms of nitrogen, boron, and hydrogen can be introduced into diamond during the growth at significant concentrations (up to atomic percents). Transition metals nickel and cobalt, which are commonly used for growth of synthetic diamond by high-pressure high-temperature techniques, have been detected in diamond as individual atoms; the maximum concentration is 0.01% for nickel[53] and even less for cobalt. Virtually any element can be introduced to diamond by ion implantation.[54]
Nitrogen is by far the most common impurity found in gem diamonds and is responsible for the yellow and brown color in diamonds. Boron is responsible for the blue color.[55] Color in diamond has two additional sources: irradiation (usually by alpha particles), that causes the color in green diamonds, and plastic deformation of the diamond crystal lattice. Plastic deformation is the cause of color in some brown[56] and perhaps pink and red diamonds.[57] In order of increasing rarity, yellow diamond is followed by brown, colorless, then by blue, green, black, pink, orange, purple, and red.[34] «Black», or carbonado, diamonds are not truly black, but rather contain numerous dark inclusions that give the gems their dark appearance. Colored diamonds contain impurities or structural defects that cause the coloration, while pure or nearly pure diamonds are transparent and colorless. Most diamond impurities replace a carbon atom in the crystal lattice, known as a carbon flaw. The most common impurity, nitrogen, causes a slight to intense yellow coloration depending upon the type and concentration of nitrogen present.[34] The Gemological Institute of America (GIA) classifies low saturation yellow and brown diamonds as diamonds in the normal color range, and applies a grading scale from «D» (colorless) to «Z» (light yellow). Yellow diamonds of high color saturation or a different color, such as pink or blue, are called fancy colored diamonds and fall under a different grading scale.[34]
In 2008, the Wittelsbach Diamond, a 35.56-carat (7.112 g) blue diamond once belonging to the King of Spain, fetched over US$24 million at a Christie’s auction.[58] In May 2009, a 7.03-carat (1.406 g) blue diamond fetched the highest price per carat ever paid for a diamond when it was sold at auction for 10.5 million Swiss francs (6.97 million euros, or US$9.5 million at the time).[59] That record was, however, beaten the same year: a 5-carat (1.0 g) vivid pink diamond was sold for $10.8 million in Hong Kong on December 1, 2009.[60]
Clarity
Clarity is one of the 4C’s (color, clarity, cut and carat weight) that helps in identifying the quality of diamonds. The Gemological Institute of America (GIA) developed 11 clarity scales to decide the quality of a diamond for its sale value. The GIA clarity scale spans from Flawless (FL) to included (I) having internally flawless (IF), very, very slightly included (VVS), very slightly included (VS) and slightly included (SI) in between. Impurities in natural diamonds are due to the presence of natural minerals and oxides. The clarity scale grades the diamond based on the color, size, location of impurity and quantity of clarity visible under 10x magnification.[61] Inclusions in diamond can be extracted by optical methods. The process is to take pre-enhancement images, identifying the inclusion removal part and finally removing the diamond facets and noises.[62]
Fluorescence
Extremely rare purple fluorescent diamonds from the Ellendale L-Channel deposit in Australia
Between 25% to 35% of natural diamonds exhibit some degree of fluorescence when examined under invisible long-wave Ultraviolet light or higher energy radiation sources such as X-rays and lasers.[63] Incandescent lighting will not cause a diamond to fluoresce. Diamonds can fluoresce in a variety of colours including blue (most common), orange, yellow, white, green and very rarely red and purple. Although the causes are not well understood, variations in the atomic structure, such as the number of nitrogen atoms present are thought to contribute to the phenomenon.
Thermal Conductivity
Diamonds can be identified by their high thermal conductivity (900–2320 W·m−1·K−1).[64] Their high refractive index is also indicative, but other materials have similar refractivity.
Geology
Diamonds are extremely rare, with concentrations of at most parts per billion in source rock.[20] Before the 20th century, most diamonds were found in alluvial deposits. Loose diamonds are also found along existing and ancient shorelines, where they tend to accumulate because of their size and density.[65]: 149 Rarely, they have been found in glacial till (notably in Wisconsin and Indiana), but these deposits are not of commercial quality.[65]: 19 These types of deposit were derived from localized igneous intrusions through weathering and transport by wind or water.[66]
Most diamonds come from the Earth’s mantle, and most of this section discusses those diamonds. However, there are other sources. Some blocks of the crust, or terranes, have been buried deep enough as the crust thickened so they experienced ultra-high-pressure metamorphism. These have evenly distributed microdiamonds that show no sign of transport by magma. In addition, when meteorites strike the ground, the shock wave can produce high enough temperatures and pressures for microdiamonds and nanodiamonds to form.[66] Impact-type microdiamonds can be used as an indicator of ancient impact craters.[67] Popigai impact structure in Russia may have the world’s largest diamond deposit, estimated at trillions of carats, and formed by an asteroid impact.[68]
A common misconception is that diamonds form from highly compressed coal. Coal is formed from buried prehistoric plants, and most diamonds that have been dated are far older than the first land plants. It is possible that diamonds can form from coal in subduction zones, but diamonds formed in this way are rare, and the carbon source is more likely carbonate rocks and organic carbon in sediments, rather than coal.[69][70]
Surface distribution
Diamonds are far from evenly distributed over the Earth. A rule of thumb known as Clifford’s rule states that they are almost always found in kimberlites on the oldest part of cratons, the stable cores of continents with typical ages of 2.5 billion years or more.[66][71]: 314 However, there are exceptions. The Argyle diamond mine in Australia, the largest producer of diamonds by weight in the world, is located in a mobile belt, also known as an orogenic belt,[72] a weaker zone surrounding the central craton that has undergone compressional tectonics. Instead of kimberlite, the host rock is lamproite. Lamproites with diamonds that are not economically viable are also found in the United States, India, and Australia.[66] In addition, diamonds in the Wawa belt of the Superior province in Canada and microdiamonds in the island arc of Japan are found in a type of rock called lamprophyre.[66]
Kimberlites can be found in narrow (1 to 4 meters) dikes and sills, and in pipes with diameters that range from about 75 m to 1.5 km. Fresh rock is dark bluish green to greenish gray, but after exposure rapidly turns brown and crumbles.[73] It is hybrid rock with a chaotic mixture of small minerals and rock fragments (clasts) up to the size of watermelons. They are a mixture of xenocrysts and xenoliths (minerals and rocks carried up from the lower crust and mantle), pieces of surface rock, altered minerals such as serpentine, and new minerals that crystallized during the eruption. The texture varies with depth. The composition forms a continuum with carbonatites, but the latter have too much oxygen for carbon to exist in a pure form. Instead, it is locked up in the mineral calcite (CaCO
3).[66]
All three of the diamond-bearing rocks (kimberlite, lamproite and lamprophyre) lack certain minerals (melilite and kalsilite) that are incompatible with diamond formation. In kimberlite, olivine is large and conspicuous, while lamproite has Ti-phlogopite and lamprophyre has biotite and amphibole. They are all derived from magma types that erupt rapidly from small amounts of melt, are rich in volatiles and magnesium oxide, and are less oxidizing than more common mantle melts such as basalt. These characteristics allow the melts to carry diamonds to the surface before they dissolve.[66]
Exploration
Diavik Mine, on an island in Lac de Gras in northern Canada
Kimberlite pipes can be difficult to find. They weather quickly (within a few years after exposure) and tend to have lower topographic relief than surrounding rock. If they are visible in outcrops, the diamonds are never visible because they are so rare. In any case, kimberlites are often covered with vegetation, sediments, soils, or lakes. In modern searches, geophysical methods such as aeromagnetic surveys, electrical resistivity, and gravimetry, help identify promising regions to explore. This is aided by isotopic dating and modeling of the geological history. Then surveyors must go to the area and collect samples, looking for kimberlite fragments or indicator minerals. The latter have compositions that reflect the conditions where diamonds form, such as extreme melt depletion or high pressures in eclogites. However, indicator minerals can be misleading; a better approach is geothermobarometry, where the compositions of minerals are analyzed as if they were in equilibrium with mantle minerals.[66]
Finding kimberlites requires persistence, and only a small fraction contain diamonds that are commercially viable. The only major discoveries since about 1980 have been in Canada. Since existing mines have lifetimes of as little as 25 years, there could be a shortage of new diamonds in the future.[66]
Ages
Diamonds are dated by analyzing inclusions using the decay of radioactive isotopes. Depending on the elemental abundances, one can look at the decay of rubidium to strontium, samarium to neodymium, uranium to lead, argon-40 to argon-39, or rhenium to osmium. Those found in kimberlites have ages ranging from 1 to 3.5 billion years, and there can be multiple ages in the same kimberlite, indicating multiple episodes of diamond formation. The kimberlites themselves are much younger. Most of them have ages between tens of millions and 300 million years old, although there are some older exceptions (Argyle, Premier and Wawa). Thus, the kimberlites formed independently of the diamonds and served only to transport them to the surface.[20][66] Kimberlites are also much younger than the cratons they have erupted through. The reason for the lack of older kimberlites is unknown, but it suggests there was some change in mantle chemistry or tectonics. No kimberlite has erupted in human history.[66]
Origin in mantle
Red garnet inclusion in a diamond[74]
Most gem-quality diamonds come from depths of 150–250 km in the lithosphere. Such depths occur below cratons in mantle keels, the thickest part of the lithosphere. These regions have high enough pressure and temperature to allow diamonds to form and they are not convecting, so diamonds can be stored for billions of years until a kimberlite eruption samples them.[66]
Host rocks in a mantle keel include harzburgite and lherzolite, two type of peridotite. The most dominant rock type in the upper mantle, peridotite is an igneous rock consisting mostly of the minerals olivine and pyroxene; it is low in silica and high in magnesium. However, diamonds in peridotite rarely survive the trip to the surface.[66] Another common source that does keep diamonds intact is eclogite, a metamorphic rock that typically forms from basalt as an oceanic plate plunges into the mantle at a subduction zone.[20]
A smaller fraction of diamonds (about 150 have been studied) come from depths of 330–660 km, a region that includes the transition zone. They formed in eclogite but are distinguished from diamonds of shallower origin by inclusions of majorite (a form of garnet with excess silicon). A similar proportion of diamonds comes from the lower mantle at depths between 660 and 800 km.[20]
Diamond is thermodynamically stable at high pressures and temperatures, with the phase transition from graphite occurring at greater temperatures as the pressure increases. Thus, underneath continents it becomes stable at temperatures of 950 degrees Celsius and pressures of 4.5 gigapascals, corresponding to depths of 150 kilometers or greater. In subduction zones, which are colder, it becomes stable at temperatures of 800 °C and pressures of 3.5 gigapascals. At depths greater than 240 km, iron-nickel metal phases are present and carbon is likely to be either dissolved in them or in the form of carbides. Thus, the deeper origin of some diamonds may reflect unusual growth environments.[20][66]
In 2018 the first known natural samples of a phase of ice called Ice VII were found as inclusions in diamond samples. The inclusions formed at depths between 400 and 800 km, straddling the upper and lower mantle, and provide evidence for water-rich fluid at these depths.[75][76]
Carbon sources
The mantle has roughly one billion gigatonnes of carbon (for comparison, the atmosphere-ocean system has about 44,000 gigatonnes).[77] Carbon has two stable isotopes, 12C and 13C, in a ratio of approximately 99:1 by mass.[66] This ratio has a wide range in meteorites, which implies that it also varied a lot in the early Earth. It can also be altered by surface processes like photosynthesis. The fraction is generally compared to a standard sample using a ratio δ13C expressed in parts per thousand. Common rocks from the mantle such as basalts, carbonatites, and kimberlites have ratios between −8 and −2. On the surface, organic sediments have an average of −25 while carbonates have an average of 0.[20]
Populations of diamonds from different sources have distributions of δ13C that vary markedly. Peridotitic diamonds are mostly within the typical mantle range; eclogitic diamonds have values from −40 to +3, although the peak of the distribution is in the mantle range. This variability implies that they are not formed from carbon that is primordial (having resided in the mantle since the Earth formed). Instead, they are the result of tectonic processes, although (given the ages of diamonds) not necessarily the same tectonic processes that act in the present.[66]
Formation and growth
Age zones in a diamond.[74]
Diamonds in the mantle form through a metasomatic process where a C-O-H-N-S fluid or melt dissolves minerals in a rock and replaces them with new minerals. (The vague term C-O-H-N-S is commonly used because the exact composition is not known.) Diamonds form from this fluid either by reduction of oxidized carbon (e.g., CO2 or CO3) or oxidation of a reduced phase such as methane.[20]
Using probes such as polarized light, photoluminescence, and cathodoluminescence, a series of growth zones can be identified in diamonds. The characteristic pattern in diamonds from the lithosphere involves a nearly concentric series of zones with very thin oscillations in luminescence and alternating episodes where the carbon is resorbed by the fluid and then grown again. Diamonds from below the lithosphere have a more irregular, almost polycrystalline texture, reflecting the higher temperatures and pressures as well as the transport of the diamonds by convection.[66]
Transport to the surface
Diagram of a volcanic pipe
Geological evidence supports a model in which kimberlite magma rises at 4–20 meters per second, creating an upward path by hydraulic fracturing of the rock. As the pressure decreases, a vapor phase exsolves from the magma, and this helps to keep the magma fluid. At the surface, the initial eruption explodes out through fissures at high speeds (over 200 m/s (450 mph)). Then, at lower pressures, the rock is eroded, forming a pipe and producing fragmented rock (breccia). As the eruption wanes, there is pyroclastic phase and then metamorphism and hydration produces serpentinites.[66]
Double diamonds
Double diamond discovered in the Ellendale Diamond Field, Western Australia
In rare cases, diamonds have been found that contain a cavity within which is a second diamond. The first double diamond, the Matryoshka, was found by Alrosa in Yakutia, Russia, in 2019.[78] Another one was found in the Ellendale Diamond Field in Western Australia in 2021.[79]
In space
Although diamonds on Earth are rare, they are very common in space. In meteorites, about three percent of the carbon is in the form of nanodiamonds, having diameters of a few nanometers. Sufficiently small diamonds can form in the cold of space because their lower surface energy makes them more stable than graphite. The isotopic signatures of some nanodiamonds indicate they were formed outside the Solar System in stars.[80]
High pressure experiments predict that large quantities of diamonds condense from methane into a «diamond rain» on the ice giant planets Uranus and Neptune.[81][82][83] Some extrasolar planets may be almost entirely composed of diamond.[84]
Diamonds may exist in carbon-rich stars, particularly white dwarfs. One theory for the origin of carbonado, the toughest form of diamond, is that it originated in a white dwarf or supernova.[85][86] Diamonds formed in stars may have been the first minerals.[87]
Industry
The most familiar uses of diamonds today are as gemstones used for adornment, and as industrial abrasives for cutting hard materials. The markets for gem-grade and industrial-grade diamonds value diamonds differently.
Gem-grade diamonds
The dispersion of white light into spectral colors is the primary gemological characteristic of gem diamonds. In the 20th century, experts in gemology developed methods of grading diamonds and other gemstones based on the characteristics most important to their value as a gem. Four characteristics, known informally as the four Cs, are now commonly used as the basic descriptors of diamonds: these are its mass in carats (a carat being equal to 0.2 grams), cut (quality of the cut is graded according to proportions, symmetry and polish), color (how close to white or colorless; for fancy diamonds how intense is its hue), and clarity (how free is it from inclusions). A large, flawless diamond is known as a paragon.[88]
A large trade in gem-grade diamonds exists. Although most gem-grade diamonds are sold newly polished, there is a well-established market for resale of polished diamonds (e.g. pawnbroking, auctions, second-hand jewelry stores, diamantaires, bourses, etc.). One hallmark of the trade in gem-quality diamonds is its remarkable concentration: wholesale trade and diamond cutting is limited to just a few locations; in 2003, 92% of the world’s diamonds were cut and polished in Surat, India.[89] Other important centers of diamond cutting and trading are the Antwerp diamond district in Belgium, where the International Gemological Institute is based, London, the Diamond District in New York City, the Diamond Exchange District in Tel Aviv and Amsterdam. One contributory factor is the geological nature of diamond deposits: several large primary kimberlite-pipe mines each account for significant portions of market share (such as the Jwaneng mine in Botswana, which is a single large-pit mine that can produce between 12,500,000 and 15,000,000 carats (2,500 and 3,000 kg) of diamonds per year[90]). Secondary alluvial diamond deposits, on the other hand, tend to be fragmented amongst many different operators because they can be dispersed over many hundreds of square kilometers (e.g., alluvial deposits in Brazil).[citation needed]
The production and distribution of diamonds is largely consolidated in the hands of a few key players, and concentrated in traditional diamond trading centers, the most important being Antwerp, where 80% of all rough diamonds, 50% of all cut diamonds and more than 50% of all rough, cut and industrial diamonds combined are handled.[91] This makes Antwerp a de facto «world diamond capital».[92] The city of Antwerp also hosts the Antwerpsche Diamantkring, created in 1929 to become the first and biggest diamond bourse dedicated to rough diamonds.[93] Another important diamond center is New York City, where almost 80% of the world’s diamonds are sold, including auction sales.[91]
The De Beers company, as the world’s largest diamond mining company, holds a dominant position in the industry, and has done so since soon after its founding in 1888 by the British businessman Cecil Rhodes. De Beers is currently the world’s largest operator of diamond production facilities (mines) and distribution channels for gem-quality diamonds. The Diamond Trading Company (DTC) is a subsidiary of De Beers and markets rough diamonds from De Beers-operated mines. De Beers and its subsidiaries own mines that produce some 40% of annual world diamond production. For most of the 20th century over 80% of the world’s rough diamonds passed through De Beers,[94] but by 2001–2009 the figure had decreased to around 45%,[95] and by 2013 the company’s market share had further decreased to around 38% in value terms and even less by volume.[96] De Beers sold off the vast majority of its diamond stockpile in the late 1990s – early 2000s[97] and the remainder largely represents working stock (diamonds that are being sorted before sale).[98] This was well documented in the press[99] but remains little known to the general public.
As a part of reducing its influence, De Beers withdrew from purchasing diamonds on the open market in 1999 and ceased, at the end of 2008, purchasing Russian diamonds mined by the largest Russian diamond company Alrosa.[100] As of January 2011, De Beers states that it only sells diamonds from the following four countries: Botswana, Namibia, South Africa and Canada.[101] Alrosa had to suspend their sales in October 2008 due to the global energy crisis,[102] but the company reported that it had resumed selling rough diamonds on the open market by October 2009.[103] Apart from Alrosa, other important diamond mining companies include BHP, which is the world’s largest mining company;[104] Rio Tinto, the owner of the Argyle (100%), Diavik (60%), and Murowa (78%) diamond mines;[105] and Petra Diamonds, the owner of several major diamond mines in Africa.
Diamond polisher in Amsterdam
Further down the supply chain, members of The World Federation of Diamond Bourses (WFDB) act as a medium for wholesale diamond exchange, trading both polished and rough diamonds. The WFDB consists of independent diamond bourses in major cutting centers such as Tel Aviv, Antwerp, Johannesburg and other cities across the US, Europe and Asia.[34] In 2000, the WFDB and The International Diamond Manufacturers Association established the World Diamond Council to prevent the trading of diamonds used to fund war and inhumane acts. WFDB’s additional activities include sponsoring the World Diamond Congress every two years, as well as the establishment of the International Diamond Council (IDC) to oversee diamond grading.[106]
Once purchased by Sightholders (which is a trademark term referring to the companies that have a three-year supply contract with DTC), diamonds are cut and polished in preparation for sale as gemstones (‘industrial’ stones are regarded as a by-product of the gemstone market; they are used for abrasives).[107] The cutting and polishing of rough diamonds is a specialized skill that is concentrated in a limited number of locations worldwide.[107] Traditional diamond cutting centers are Antwerp, Amsterdam, Johannesburg, New York City, and Tel Aviv. Recently, diamond cutting centers have been established in China, India, Thailand, Namibia and Botswana.[107] Cutting centers with lower cost of labor, notably Surat in Gujarat, India, handle a larger number of smaller carat diamonds, while smaller quantities of larger or more valuable diamonds are more likely to be handled in Europe or North America. The recent expansion of this industry in India, employing low cost labor, has allowed smaller diamonds to be prepared as gems in greater quantities than was previously economically feasible.[91]
Diamonds prepared as gemstones are sold on diamond exchanges called bourses. There are 28 registered diamond bourses in the world.[108] Bourses are the final tightly controlled step in the diamond supply chain; wholesalers and even retailers are able to buy relatively small lots of diamonds at the bourses, after which they are prepared for final sale to the consumer. Diamonds can be sold already set in jewelry, or sold unset («loose»). According to the Rio Tinto, in 2002 the diamonds produced and released to the market were valued at US$9 billion as rough diamonds, US$14 billion after being cut and polished, US$28 billion in wholesale diamond jewelry, and US$57 billion in retail sales.[109]
Cutting
The Daria-i-Noor Diamond—an example of unusual diamond cut and jewelry arrangement.
Mined rough diamonds are converted into gems through a multi-step process called «cutting». Diamonds are extremely hard, but also brittle and can be split up by a single blow. Therefore, diamond cutting is traditionally considered as a delicate procedure requiring skills, scientific knowledge, tools and experience. Its final goal is to produce a faceted jewel where the specific angles between the facets would optimize the diamond luster, that is dispersion of white light, whereas the number and area of facets would determine the weight of the final product. The weight reduction upon cutting is significant and can be of the order of 50%.[110] Several possible shapes are considered, but the final decision is often determined not only by scientific, but also practical considerations. For example, the diamond might be intended for display or for wear, in a ring or a necklace, singled or surrounded by other gems of certain color and shape.[111] Some of them may be considered as classical, such as round, pear, marquise, oval, hearts and arrows diamonds, etc. Some of them are special, produced by certain companies, for example, Phoenix, Cushion, Sole Mio diamonds, etc.[112]
The most time-consuming part of the cutting is the preliminary analysis of the rough stone. It needs to address a large number of issues, bears much responsibility, and therefore can last years in case of unique diamonds. The following issues are considered:
- The hardness of diamond and its ability to cleave strongly depend on the crystal orientation. Therefore, the crystallographic structure of the diamond to be cut is analyzed using X-ray diffraction to choose the optimal cutting directions.
- Most diamonds contain visible non-diamond inclusions and crystal flaws. The cutter has to decide which flaws are to be removed by the cutting and which could be kept.
- The diamond can be split by a single, well calculated blow of a hammer to a pointed tool, which is quick, but risky. Alternatively, it can be cut with a diamond saw, which is a more reliable but tedious procedure.[111][113]
After initial cutting, the diamond is shaped in numerous stages of polishing. Unlike cutting, which is a responsible but quick operation, polishing removes material by gradual erosion and is extremely time-consuming. The associated technique is well developed; it is considered as a routine and can be performed by technicians.[114] After polishing, the diamond is reexamined for possible flaws, either remaining or induced by the process. Those flaws are concealed through various diamond enhancement techniques, such as repolishing, crack filling, or clever arrangement of the stone in the jewelry. Remaining non-diamond inclusions are removed through laser drilling and filling of the voids produced.[29]
Marketing
Diamond Balance Scale 0.01 — 25 Carat Jewelers Measuring Tool
Marketing has significantly affected the image of diamond as a valuable commodity.
N. W. Ayer & Son, the advertising firm retained by De Beers in the mid-20th century, succeeded in reviving the American diamond market and the firm created new markets in countries where no diamond tradition had existed before. N. W. Ayer’s marketing included product placement, advertising focused on the diamond product itself rather than the De Beers brand, and associations with celebrities and royalty. Without advertising the De Beers brand, De Beers was advertising its competitors’ diamond products as well,[115] but this was not a concern as De Beers dominated the diamond market throughout the 20th century. De Beers’ market share dipped temporarily to second place in the global market below Alrosa in the aftermath of the global economic crisis of 2008, down to less than 29% in terms of carats mined, rather than sold.[116] The campaign lasted for decades but was effectively discontinued by early 2011. De Beers still advertises diamonds, but the advertising now mostly promotes its own brands, or licensed product lines, rather than completely «generic» diamond products.[116] The campaign was perhaps best captured by the slogan «a diamond is forever».[117] This slogan is now being used by De Beers Diamond Jewelers,[118] a jewelry firm which is a 50/50% joint venture between the De Beers mining company and LVMH, the luxury goods conglomerate.
Brown-colored diamonds constituted a significant part of the diamond production, and were predominantly used for industrial purposes. They were seen as worthless for jewelry (not even being assessed on the diamond color scale). After the development of Argyle diamond mine in Australia in 1986, and marketing, brown diamonds have become acceptable gems.[119][120] The change was mostly due to the numbers: the Argyle mine, with its 35,000,000 carats (7,000 kg) of diamonds per year, makes about one-third of global production of natural diamonds;[121] 80% of Argyle diamonds are brown.[122]
Industrial-grade diamonds
A scalpel with synthetic diamond blade
Close-up photograph of an angle grinder blade with tiny diamonds shown embedded in the metal
A diamond knife blade used for cutting ultrathin sections (typically 70 to 350 nm) for transmission electron microscopy
Industrial diamonds are valued mostly for their hardness and thermal conductivity, making many of the gemological characteristics of diamonds, such as the 4 Cs, irrelevant for most applications. Eighty percent of mined diamonds (equal to about 135,000,000 carats (27,000 kg) annually) are unsuitable for use as gemstones and are used industrially.[123] In addition to mined diamonds, synthetic diamonds found industrial applications almost immediately after their invention in the 1950s; in 2014, 4,500,000,000 carats (900,000 kg) of synthetic diamonds were produced, 90% of which were produced in China. Approximately 90% of diamond grinding grit is currently of synthetic origin.[124]
The boundary between gem-quality diamonds and industrial diamonds is poorly defined and partly depends on market conditions (for example, if demand for polished diamonds is high, some lower-grade stones will be polished into low-quality or small gemstones rather than being sold for industrial use). Within the category of industrial diamonds, there is a sub-category comprising the lowest-quality, mostly opaque stones, which are known as bort.[125]
Industrial use of diamonds has historically been associated with their hardness, which makes diamond the ideal material for cutting and grinding tools. As the hardest known naturally occurring material, diamond can be used to polish, cut, or wear away any material, including other diamonds. Common industrial applications of this property include diamond-tipped drill bits and saws, and the use of diamond powder as an abrasive. Less expensive industrial-grade diamonds (bort) with more flaws and poorer color than gems, are used for such purposes.[126] Diamond is not suitable for machining ferrous alloys at high speeds, as carbon is soluble in iron at the high temperatures created by high-speed machining, leading to greatly increased wear on diamond tools compared to alternatives.[127]
Specialized applications include use in laboratories as containment for high-pressure experiments (see diamond anvil cell), high-performance bearings, and limited use in specialized windows.[125] With the continuing advances being made in the production of synthetic diamonds, future applications are becoming feasible. The high thermal conductivity of diamond makes it suitable as a heat sink for integrated circuits in electronics.[128]
Mining
Approximately 130,000,000 carats (26,000 kg) of diamonds are mined annually, with a total value of nearly US$9 billion, and about 100,000 kg (220,000 lb) are synthesized annually.[129]
Roughly 49% of diamonds originate from Central and Southern Africa, although significant sources of the mineral have been discovered in Canada, India, Russia, Brazil, and Australia.[124] They are mined from kimberlite and lamproite volcanic pipes, which can bring diamond crystals, originating from deep within the Earth where high pressures and temperatures enable them to form, to the surface. The mining and distribution of natural diamonds are subjects of frequent controversy such as concerns over the sale of blood diamonds or conflict diamonds by African paramilitary groups.[130] The diamond supply chain is controlled by a limited number of powerful businesses, and is also highly concentrated in a small number of locations around the world.
Only a very small fraction of the diamond ore consists of actual diamonds. The ore is crushed, during which care is required not to destroy larger diamonds, and then sorted by density. Today, diamonds are located in the diamond-rich density fraction with the help of X-ray fluorescence, after which the final sorting steps are done by hand. Before the use of X-rays became commonplace,[110] the separation was done with grease belts; diamonds have a stronger tendency to stick to grease than the other minerals in the ore.[34]
Historically, diamonds were found only in alluvial deposits in Guntur and Krishna district of the Krishna River delta in Southern India.[131] India led the world in diamond production from the time of their discovery in approximately the 9th century BC[132][133] to the mid-18th century AD, but the commercial potential of these sources had been exhausted by the late 18th century and at that time India was eclipsed by Brazil where the first non-Indian diamonds were found in 1725.[132] Currently, one of the most prominent Indian mines is located at Panna.[134]
Diamond extraction from primary deposits (kimberlites and lamproites) started in the 1870s after the discovery of the Diamond Fields in South Africa.[135] Production has increased over time and now an accumulated total of 4,500,000,000 carats (900,000 kg) have been mined since that date.[136] Twenty percent of that amount has been mined in the last five years, and during the last 10 years, nine new mines have started production; four more are waiting to be opened soon. Most of these mines are located in Canada, Zimbabwe, Angola, and one in Russia.[136]
In the U.S., diamonds have been found in Arkansas, Colorado, New Mexico, Wyoming, and Montana.[137][138] In 2004, the discovery of a microscopic diamond in the U.S. led to the January 2008 bulk-sampling of kimberlite pipes in a remote part of Montana. The Crater of Diamonds State Park in Arkansas is open to the public, and is the only mine in the world where members of the public can dig for diamonds.[138]
Today, most commercially viable diamond deposits are in Russia (mostly in Sakha Republic, for example Mir pipe and Udachnaya pipe), Botswana, Australia (Northern and Western Australia) and the Democratic Republic of the Congo.[139] In 2005, Russia produced almost one-fifth of the global diamond output, according to the British Geological Survey. Australia boasts the richest diamantiferous pipe, with production from the Argyle diamond mine reaching peak levels of 42 metric tons per year in the 1990s.[137][140] There are also commercial deposits being actively mined in the Northwest Territories of Canada and Brazil.[124] Diamond prospectors continue to search the globe for diamond-bearing kimberlite and lamproite pipes.
Political issues
In some of the more politically unstable central African and west African countries, revolutionary groups have taken control of diamond mines, using proceeds from diamond sales to finance their operations. Diamonds sold through this process are known as conflict diamonds or blood diamonds.[130]
In response to public concerns that their diamond purchases were contributing to war and human rights abuses in central and western Africa, the United Nations, the diamond industry and diamond-trading nations introduced the Kimberley Process in 2002.[141] The Kimberley Process aims to ensure that conflict diamonds do not become intermixed with the diamonds not controlled by such rebel groups. This is done by requiring diamond-producing countries to provide proof that the money they make from selling the diamonds is not used to fund criminal or revolutionary activities. Although the Kimberley Process has been moderately successful in limiting the number of conflict diamonds entering the market, some still find their way in. According to the International Diamond Manufacturers Association, conflict diamonds constitute 2–3% of all diamonds traded.[142] Two major flaws still hinder the effectiveness of the Kimberley Process: (1) the relative ease of smuggling diamonds across African borders, and (2) the violent nature of diamond mining in nations that are not in a technical state of war and whose diamonds are therefore considered «clean».[141]
The Canadian Government has set up a body known as the Canadian Diamond Code of Conduct[143] to help authenticate Canadian diamonds. This is a stringent tracking system of diamonds and helps protect the «conflict free» label of Canadian diamonds.[144]
Mineral resource exploitation in general causes irreversible environmental damage, which must be weighed against the socio-economic benefits to a country.[145]
Synthetics, simulants, and enhancements
Synthetics
Synthetic diamonds are diamonds manufactured in a laboratory, as opposed to diamonds mined from the Earth. The gemological and industrial uses of diamond have created a large demand for rough stones. This demand has been satisfied in large part by synthetic diamonds, which have been manufactured by various processes for more than half a century. However, in recent years it has become possible to produce gem-quality synthetic diamonds of significant size.[65] It is possible to make colorless synthetic gemstones that, on a molecular level, are identical to natural stones and so visually similar that only a gemologist with special equipment can tell the difference.[146]
The majority of commercially available synthetic diamonds are yellow and are produced by so-called high-pressure high-temperature (HPHT) processes.[147] The yellow color is caused by nitrogen impurities. Other colors may also be reproduced such as blue, green or pink, which are a result of the addition of boron or from irradiation after synthesis.[148]
Another popular method of growing synthetic diamond is chemical vapor deposition (CVD). The growth occurs under low pressure (below atmospheric pressure). It involves feeding a mixture of gases (typically 1 to 99 methane to hydrogen) into a chamber and splitting them into chemically active radicals in a plasma ignited by microwaves, hot filament, arc discharge, welding torch, or laser.[149] This method is mostly used for coatings, but can also produce single crystals several millimeters in size (see picture).[129]
As of 2010, nearly all 5,000 million carats (1,000 tonnes) of synthetic diamonds produced per year are for industrial use. Around 50% of the 133 million carats of natural diamonds mined per year end up in industrial use.[146][150] Mining companies’ expenses average 40 to 60 US dollars per carat for natural colorless diamonds, while synthetic manufacturers’ expenses average $2,500 per carat for synthetic, gem-quality colorless diamonds.[146]: 79 However, a purchaser is more likely to encounter a synthetic when looking for a fancy-colored diamond because nearly all synthetic diamonds are fancy-colored, while only 0.01% of natural diamonds are.[151]
-
Synthetic diamonds of various colors grown by the high-pressure high-temperature technique
-
Colorless gem cut from diamond grown by chemical vapor deposition
Simulants
Gem-cut synthetic silicon carbide set in a ring
A diamond simulant is a non-diamond material that is used to simulate the appearance of a diamond, and may be referred to as diamante. Cubic zirconia is the most common. The gemstone moissanite (silicon carbide) can be treated as a diamond simulant, though more costly to produce than cubic zirconia. Both are produced synthetically.[152]
Enhancements
Diamond enhancements are specific treatments performed on natural or synthetic diamonds (usually those already cut and polished into a gem), which are designed to better the gemological characteristics of the stone in one or more ways. These include laser drilling to remove inclusions, application of sealants to fill cracks, treatments to improve a white diamond’s color grade, and treatments to give fancy color to a white diamond.[153]
Coatings are increasingly used to give a diamond simulant such as cubic zirconia a more «diamond-like» appearance. One such substance is diamond-like carbon—an amorphous carbonaceous material that has some physical properties similar to those of the diamond. Advertising suggests that such a coating would transfer some of these diamond-like properties to the coated stone, hence enhancing the diamond simulant. Techniques such as Raman spectroscopy should easily identify such a treatment.[154]
Identification
Early diamond identification tests included a scratch test relying on the superior hardness of diamond. This test is destructive, as a diamond can scratch another diamond, and is rarely used nowadays. Instead, diamond identification relies on its superior thermal conductivity. Electronic thermal probes are widely used in the gemological centers to separate diamonds from their imitations. These probes consist of a pair of battery-powered thermistors mounted in a fine copper tip. One thermistor functions as a heating device while the other measures the temperature of the copper tip: if the stone being tested is a diamond, it will conduct the tip’s thermal energy rapidly enough to produce a measurable temperature drop. This test takes about two to three seconds.[155]
Whereas the thermal probe can separate diamonds from most of their simulants, distinguishing between various types of diamond, for example synthetic or natural, irradiated or non-irradiated, etc., requires more advanced, optical techniques. Those techniques are also used for some diamonds simulants, such as silicon carbide, which pass the thermal conductivity test. Optical techniques can distinguish between natural diamonds and synthetic diamonds. They can also identify the vast majority of treated natural diamonds.[156] «Perfect» crystals (at the atomic lattice level) have never been found, so both natural and synthetic diamonds always possess characteristic imperfections, arising from the circumstances of their crystal growth, that allow them to be distinguished from each other.[157]
Laboratories use techniques such as spectroscopy, microscopy, and luminescence under shortwave ultraviolet light to determine a diamond’s origin.[156] They also use specially made instruments to aid them in the identification process. Two screening instruments are the DiamondSure and the DiamondView, both produced by the DTC and marketed by the GIA.[158]
Several methods for identifying synthetic diamonds can be performed, depending on the method of production and the color of the diamond. CVD diamonds can usually be identified by an orange fluorescence. D-J colored diamonds can be screened through the Swiss Gemmological Institute’s[159] Diamond Spotter. Stones in the D-Z color range can be examined through the DiamondSure UV/visible spectrometer, a tool developed by De Beers.[157] Similarly, natural diamonds usually have minor imperfections and flaws, such as inclusions of foreign material, that are not seen in synthetic diamonds.
Screening devices based on diamond type detection can be used to make a distinction between diamonds that are certainly natural and diamonds that are potentially synthetic. Those potentially synthetic diamonds require more investigation in a specialized lab. Examples of commercial screening devices are D-Screen (WTOCD / HRD Antwerp), Alpha Diamond Analyzer (Bruker / HRD Antwerp), and D-Secure (DRC Techno).
Etymology, earliest use and composition discovery
The name diamond is derived from Ancient Greek: ἀδάμας (adámas), ‘proper, unalterable, unbreakable, untamed’, from ἀ- (a-), ‘not’ + Ancient Greek: δαμάω (damáō), ‘to overpower, tame’.[160] Diamonds are thought to have been first recognized and mined in India, where significant alluvial deposits of the stone could be found many centuries ago along the rivers Penner, Krishna, and Godavari. Diamonds have been known in India for at least 3,000 years but most likely 6,000 years.[132]
Diamonds have been treasured as gemstones since their use as religious icons in ancient India. Their usage in engraving tools also dates to early human history.[161][162] The popularity of diamonds has risen since the 19th century because of increased supply, improved cutting and polishing techniques, growth in the world economy, and innovative and successful advertising campaigns.[117]
In 1772, the French scientist Antoine Lavoisier used a lens to concentrate the rays of the sun on a diamond in an atmosphere of oxygen, and showed that the only product of the combustion was carbon dioxide, proving that diamond is composed of carbon.[163] Later in 1797, the English chemist Smithson Tennant repeated and expanded that experiment.[164] By demonstrating that burning diamond and graphite releases the same amount of gas, he established the chemical equivalence of these substances.[30]
See also
- Deep carbon cycle
- Diamondoid
- List of diamonds
- List of largest rough diamonds
- List of minerals
- Superhard material
Citations
- ^ Warr LN (2021). «IMA–CNMNC approved mineral symbols». Mineralogical Magazine. 85 (3): 291–320. Bibcode:2021MinM…85..291W. doi:10.1180/mgm.2021.43. S2CID 235729616.
- ^ a b «Diamond». Mindat. Retrieved July 7, 2009.
- ^ «Diamond». WebMineral. Retrieved July 7, 2009.
- ^ Delhaes P (2000). «Polymorphism of carbon». In Delhaes P (ed.). Graphite and precursors. Gordon & Breach. pp. 1–24. ISBN 978-90-5699-228-6.
- ^ Pierson HO (2012). Handbook of carbon, graphite, diamond, and fullerenes: properties, processing, and applications. Noyes Publications. pp. 40–41. ISBN 978-0-8155-1739-9.
- ^ Angus JC (1997). «Structure and thermochemistry of diamond». In Paoletti A, Tucciarone A (eds.). The physics of diamond. IOS Press. pp. 9–30. ISBN 978-1-61499-220-2.
- ^ a b Rock PA (1983). Chemical Thermodynamics. University Science Books. pp. 257–260. ISBN 978-1-891389-32-0.
- ^ Gray T (October 8, 2009). «Gone in a Flash». Popular Science. Retrieved October 31, 2018.
- ^ Chen Y, Zhang L (2013). Polishing of diamond materials: mechanisms, modeling and implementation. Springer Science & Business Media. pp. 1–2. ISBN 978-1-84996-408-1.
- ^ a b Bundy P, Bassett WA, Weathers MS, Hemley RJ, Mao HK, Goncharov AF (1996). «The pressure-temperature phase and transformation diagram for carbon; updated through 1994». Carbon. 34 (2): 141–153. doi:10.1016/0008-6223(96)00170-4.
- ^ Wang CX, Yang GW (2012). «Thermodynamic and kinetic approaches of diamond and related nanomaterials formed by laser ablation in liquid». In Yang G (ed.). Laser ablation in liquids: principles and applications in the preparation of nanomaterials. Pan Stanford Pub. pp. 164–165. ISBN 978-981-4241-52-6.
- ^ Wang X, Scandolo S, Car R (October 2005). «Carbon phase diagram from ab initio molecular dynamics». Physical Review Letters. 95 (18): 185701. Bibcode:2005PhRvL..95r5701W. doi:10.1103/PhysRevLett.95.185701. PMID 16383918.
- ^ Correa AA, Bonev SA, Galli G (January 2006). «Carbon under extreme conditions: phase boundaries and electronic properties from first-principles theory». Proceedings of the National Academy of Sciences of the United States of America. 103 (5): 1204–1208. Bibcode:2006PNAS..103.1204C. doi:10.1073/pnas.0510489103. PMC 1345714. PMID 16432191.
- ^ Bland E (January 15, 2010). «Diamond oceans possible on Uranus, Neptune». Discovery News. Archived from the original on 2012-03-11. Retrieved January 16, 2010.
- ^ Silvera I (2010). «Diamond: Molten under pressure». Nature Physics. 6 (1): 9–10. Bibcode:2010NatPh…6….9S. doi:10.1038/nphys1491. S2CID 120836330.
- ^ Rajendran V (2004). Materials science. Tata McGraw-Hill Pub. p. 2.16. ISBN 978-0-07-058369-6.
- ^ a b Ashcroft NW, Mermin ND (1976). Solid state physics. Holt, Rinehart and Winston. p. 76. ISBN 978-0-03-083993-1.
- ^ Bandosz TJ, Biggs MJ, Gubbins KE, Hattori Y, Iiyama T, Kaneko T, Pikunic J, Thomson K (2003). «Molecular models of porous carbons». In Radovic LR (ed.). Chemistry and physics of carbon. Vol. 28. Marcel Dekker. pp. 46–47. ISBN 978-0-8247-0987-7.
- ^ Webster R, Read PG (2000). Gems: Their sources, descriptions and identification (5th ed.). Great Britain: Butterworth-Heinemann. p. 17. ISBN 978-0-7506-1674-4.
- ^ a b c d e f g h i j Cartigny P, Palot M, Thomassot E, Harris JW (May 30, 2014). «Diamond Formation: A Stable Isotope Perspective». Annual Review of Earth and Planetary Sciences. 42 (1): 699–732. Bibcode:2014AREPS..42..699C. doi:10.1146/annurev-earth-042711-105259.
- ^ Fukura S, Nakagawa T, Kagi H (November 2005). «High spatial resolution photoluminescence and Raman spectroscopic measurements of a natural polycrystalline diamond, carbonado». Diamond and Related Materials. 14 (11–12): 1950–1954. Bibcode:2005DRM….14.1950F. doi:10.1016/j.diamond.2005.08.046.
- ^ Mohammad G, Siddiquei MM, Abu El-Asrar AM (2006). «Poly (ADP-ribose) polymerase mediates diabetes-induced retinal neuropathy». Mediators of Inflammation. 2013 (2): 510451. arXiv:physics/0608014. Bibcode:2006ApJ…653L.153G. doi:10.1086/510451. PMC 3857786. PMID 24347828. S2CID 59405368.
- ^ «Diamonds from Outer Space: Geologists Discover Origin of Earth’s Mysterious Black Diamonds». National Science Foundation. January 8, 2007. Retrieved October 28, 2007.
- ^ «Diamonds Are Indestructible, Right?». Dominion Jewelers. 2015-12-16. Retrieved 2020-10-31.
- ^ M. Seal, «The abrasion of diamond», Proceedings of the Royal Society A 248:1254 (25 November 1958) doi:10.1098/rspa.1958.0250
- ^ Weiler HD (2021-04-13) [1954], The wear and care of records and styli, retrieved 2022-01-01 – via Shure
- ^ Neves AJ, Nazaré MH (2001). Properties, Growth and Applications of Diamond. Institution of Engineering and Technology. pp. 142–147. ISBN 978-0-85296-785-0.
- ^ Boser U (2008). «Diamonds on Demand». Smithsonian. 39 (3): 52–59.
- ^ a b Read PG (2005). Gemmology. Butterworth-Heinemann. pp. 165–166. ISBN 978-0-7506-6449-3.
- ^ a b Hazen RM (1999). The diamond makers. Cambridge University Press. pp. 7–10. ISBN 978-0-521-65474-6.
- ^ O’Donoghue M (1997). Synthetic, Imitation and Treated Gemstones. Gulf Professional Publishing. pp. 34–37. ISBN 978-0-7506-3173-0.
- ^ Lee J, Novikov NV (2005). Innovative superhard materials and sustainable coatings for advanced manufacturing. Springer. p. 102. ISBN 978-0-8493-3512-9.
- ^ Marinescu ID, Tönshoff HK, Inasaki I (2000). Handbook of ceramic grinding and polishing. William Andrew. p. 21. ISBN 978-0-8155-1424-4.
- ^ a b c d e f Harlow GE (1998). The nature of diamonds. Cambridge University Press. pp. 223, 230–249. ISBN 978-0-521-62935-5.
- ^ Eremets MI, Trojan IA, Gwaze P, Huth J, Boehler R, Blank VD (October 3, 2005). «The strength of diamond». Applied Physics Letters. 87 (14): 141902. Bibcode:2005ApPhL..87n1902E. doi:10.1063/1.2061853.
- ^ a b Dubrovinsky L, Dubrovinskaia N, Prakapenka VB, Abakumov AM (October 23, 2012). «Implementation of micro-ball nanodiamond anvils for high-pressure studies above 6 Mbar». Nature Communications. 3 (1): 1163. Bibcode:2012NatCo…3.1163D. doi:10.1038/ncomms2160. PMC 3493652. PMID 23093199.
- ^ a b Wogan T (November 2, 2012). «Improved diamond anvil cell allows higher pressures than ever before». Physics World. Nature Communications. Retrieved 2022-07-01.
- ^ Dang C, Chou JP, Dai B, Chou CT, Yang Y, Fan R, et al. (January 2021). «Achieving large uniform tensile elasticity in microfabricated diamond». Science. 371 (6524): 76–78. Bibcode:2021Sci…371…76D. doi:10.1126/science.abc4174. PMID 33384375.
- ^ Banerjee A, Bernoulli D, Zhang H, Yuen MF, Liu J, Dong J, et al. (April 2018). «Ultralarge elastic deformation of nanoscale diamond». Science. 360 (6386): 300–302. Bibcode:2018Sci…360..300B. doi:10.1126/science.aar4165. PMID 29674589.
- ^ LLorca J (April 2018). «On the quest for the strongest materials». Science. 360 (6386): 264–265. arXiv:2105.05099. Bibcode:2018Sci…360..264L. doi:10.1126/science.aat5211. PMID 29674578. S2CID 4986592.
- ^ Collins AT (1993). «The Optical and Electronic Properties of Semiconducting Diamond». Philosophical Transactions of the Royal Society A. 342 (1664): 233–244. Bibcode:1993RSPTA.342..233C. doi:10.1098/rsta.1993.0017. S2CID 202574625.
- ^ Landstrass MI, Ravi KV (1989). «Resistivity of chemical vapor deposited diamond films». Applied Physics Letters. 55 (10): 975–977. Bibcode:1989ApPhL..55..975L. doi:10.1063/1.101694.
- ^ Zhang W, Ristein J, Ley L (October 2008). «Hydrogen-terminated diamond electrodes. II. Redox activity». Physical Review. E, Statistical, Nonlinear, and Soft Matter Physics. 78 (4 Pt 1): 041603. Bibcode:2008PhRvE..78d1603Z. doi:10.1103/PhysRevE.78.041603. PMID 18999435.
- ^ Shi Z, Dao M, Tsymbalov E, Shapeev A, Li J, Suresh S (October 2020). «Metallization of diamond». Proceedings of the National Academy of Sciences of the United States of America. 117 (40): 24634–24639. Bibcode:2020PNAS..11724634S. doi:10.1073/pnas.2013565117. PMC 7547227. PMID 33020306.
- ^ Irving M (2022-04-28). «Two-inch diamond wafers could store a billion Blu-Ray’s worth of data». New Atlas. Retrieved 2022-04-29.
- ^ Wissner-Gross AD, Kaxiras E (August 2007). «Diamond stabilization of ice multilayers at human body temperature» (PDF). Physical Review. E, Statistical, Nonlinear, and Soft Matter Physics. 76 (2 Pt 1): 020501. Bibcode:2007PhRvE..76b0501W. doi:10.1103/physreve.76.020501. PMID 17929997. S2CID 44344503. Archived (PDF) from the original on 2011-07-24.
- ^ Fujimoto A, Yamada Y, Koinuma M, Sato S (June 2016). «Origins of sp(3)C peaks in C1s X-ray Photoelectron Spectra of Carbon Materials». Analytical Chemistry. 88 (12): 6110–6114. doi:10.1021/acs.analchem.6b01327. PMID 27264720.
- ^ Bauer M (2012). Precious Stones. Vol. 1. Dover Publications. pp. 115–117. ISBN 978-0-486-15125-0.
- ^ «Diamond Care and Cleaning Guide». Gemological Institute of America. Retrieved 1 August 2019.
- ^ Jones C (27 August 2016). «Diamonds are Flammable! How to Safeguard Your Jewelry». DMIA. Retrieved 1 August 2019.
- ^ Baird CS. «Can you light diamond on fire?». Science Questions with Surprising Answers. Retrieved 1 August 2019.
- ^ Lederle F, Koch J, Hübner EG (February 21, 2019). «Colored Sparks». European Journal of Inorganic Chemistry. 2019 (7): 928–937. doi:10.1002/ejic.201801300. S2CID 104449284.
- ^ Collins AT, Kanda H, Isoya J, Ammerlaan CA, Van Wyk JA (1998). «Correlation between optical absorption and EPR in high-pressure diamond grown from a nickel solvent catalyst». Diamond and Related Materials. 7 (2–5): 333–338. Bibcode:1998DRM…..7..333C. doi:10.1016/S0925-9635(97)00270-7.
- ^ Zaitsev AM (2000). «Vibronic spectra of impurity-related optical centers in diamond». Physical Review B. 61 (19): 12909–12922. Bibcode:2000PhRvB..6112909Z. doi:10.1103/PhysRevB.61.12909.
- ^ Walker J (1979). «Optical absorption and luminescence in diamond» (PDF). Reports on Progress in Physics. 42 (10): 1605–1659. Bibcode:1979RPPh…42.1605W. CiteSeerX 10.1.1.467.443. doi:10.1088/0034-4885/42/10/001. S2CID 250857323. Archived (PDF) from the original on 2015-09-06.
- ^ Hounsome LS, Jones R, Shaw MJ, Briddon PR, Öberg S, Briddon P, Öberg S (2006). «Origin of brown coloration in diamond». Physical Review B. 73 (12): 125203. Bibcode:2006PhRvB..73l5203H. doi:10.1103/PhysRevB.73.125203.
- ^ Wise RW (2001). Secrets Of The Gem Trade, The Connoisseur’s Guide To Precious Gemstones. Brunswick House Press. pp. 223–224. ISBN 978-0-9728223-8-1.
- ^ Khan U (December 10, 2008). «Blue-grey diamond belonging to King of Spain has sold for record 16.3 GBP». The Daily Telegraph. London. Retrieved March 31, 2010.
- ^
Nebehay S (May 12, 2009). «Rare blue diamond sells for record $9.5 million». Reuters. Retrieved May 13, 2009. - ^ Pomfret J (December 1, 2009). «Vivid pink diamond sells for record $10.8 million». Reuters.
- ^ Cowing MD (2014). «Objective ciamond clarity grading» (PDF). Journal of Gemmology. 34 (4): 316–332. doi:10.15506/JoG.2014.34.4.316. Archived (PDF) from the original on 2021-04-18. Retrieved 19 September 2021.
- ^ Wang W, Cai L (September 2019). «Inclusion extraction from diamond clarity images based on the analysis of diamond optical properties». Optics Express. 27 (19): 27242–27255. Bibcode:2019OExpr..2727242W. doi:10.1364/OE.27.027242. PMID 31674589. S2CID 203141270.
- ^ «Fact Checking Diamond Fluorescence: 11 Myths Dispelled». GIA 4Cs. 2018-03-27. Retrieved 2022-06-06.
- ^ Wei L, Kuo PK, Thomas RL, Anthony TR, Banholzer WF (June 1993). «Thermal conductivity of isotopically modified single crystal diamond». Physical Review Letters. 70 (24): 3764–3767. Bibcode:1993PhRvL..70.3764W. doi:10.1103/PhysRevLett.70.3764. PMID 10053956.
- ^ a b c Erlich EI, Hausel WD (2002). Diamond deposits: origin, exploration, and history of discovery. Littleton, CO: Society for Mining, Metallurgy, and Exploration. ISBN 978-0-87335-213-0.
- ^ a b c d e f g h i j k l m n o p q r Shirey SB, Shigley JE (December 1, 2013). «Recent Advances in Understanding the Geology of Diamonds». Gems & Gemology. 49 (4): 188–222. doi:10.5741/GEMS.49.4.188.
- ^ Carlson RW (2005). The Mantle and Core. Elsevier. p. 248. ISBN 978-0-08-044848-0.
- ^ Deutsch A, Masaitis VL, Langenhorst F, Grieve RA (2000). «Popigai, Siberia—well preserved giant impact structure, national treasury, and world’s geological heritage». Episodes. 23 (1): 3–12. doi:10.18814/epiiugs/2000/v23i1/002.
- ^ King H (2012). «How do diamonds form? They don’t form from coal!». Geology and Earth Science News and Information. geology.com. Archived from the original on October 30, 2013. Retrieved June 29, 2012.
- ^ Pak-Harvey A (October 31, 2013). «10 common scientific misconceptions». The Christian Science Monitor. Retrieved August 30, 2017.
- ^ Pohl WL (2011). Economic Geology: Principles and Practice. John Wiley & Sons. ISBN 978-1-4443-9486-3.
- ^ Allaby M (2013). «mobile belt». A dictionary of geology and earth sciences (4th ed.). Oxford: Oxford University Press. ISBN 978-0-19-174433-4.
- ^ Kjarsgaard BA (2007). «Kimberlite pipe models: significance for exploration» (PDF). In Milkereit B (ed.). Proceedings of Exploration 07: Fifth Decennial International Conference on Mineral Exploration. Decennial Mineral Exploration Conferences, 2007. pp. 667–677. Archived (PDF) from the original on 2012-12-24. Retrieved March 1, 2018.
- ^ a b Deep Carbon Observatory (2019). Deep Carbon Observatory: A Decade of Discovery. Washington, DC. doi:10.17863/CAM.44064. Archived from the original on 17 December 2019. Retrieved 13 December 2019.
- ^ Cartier K (April 2, 2018). «Diamond Impurities Reveal Water Deep Within the Mantle». Eos. 99. doi:10.1029/2018EO095949.
- ^ Perkins S (March 8, 2018). «Pockets of water may lie deep below Earth’s surface». Science.
- ^ Lee CA, Jiang H, Dasgupta R, Torres M (2019). «A Framework for Understanding Whole-Earth Carbon Cycling». In Orcutt BN, Daniel I, Dasgupta R (eds.). Deep carbon: past to present. Cambridge University Press. pp. 313–357. doi:10.1017/9781108677950.011. ISBN 978-1-108-67795-0. S2CID 210787128.
- ^ Wei-Haas M (2019-10-10). «Bizarre ‘nesting doll’ diamond found inside another diamond». National Geographic. Retrieved 2021-11-27.
- ^ Fowler C (2021-11-26). «Rare ‘double diamond’ discovery comes as race to restart mothballed Ellendale mine heats up». Australian Broadcasting Corporation. Retrieved 2021-11-27.
- ^ Tielens AG (July 12, 2013). «The molecular universe». Reviews of Modern Physics. 85 (3): 1021–1081. Bibcode:2013RvMP…85.1021T. doi:10.1103/RevModPhys.85.1021.
- ^ Kerr RA (October 1999). «Neptune may crush methane into diamonds». Science. 286 (5437): 25. doi:10.1126/science.286.5437.25a. PMID 10532884. S2CID 42814647.
- ^ Scandolo S, Jeanloz R (November–December 2003). «The Centers of Planets: In laboratories and computers, shocked and squeezed matter turns metallic, coughs up diamonds and reveals Earth’s white-hot center». American Scientist. 91 (6): 516–525. Bibcode:2003AmSci..91..516S. doi:10.1511/2003.38.905. JSTOR 27858301. S2CID 120975663.
- ^ Kaplan S (August 25, 2017). «It rains solid diamonds on Uranus and Neptune». The Washington Post. Retrieved October 16, 2017.
- ^ Max Planck Institute for Radio Astronomy (August 25, 2011). «A planet made of diamond». Astronomy magazine. Retrieved September 25, 2017.
- ^ Heaney PJ, de Vicenzi EP (2005). «Strange Diamonds: the Mysterious Origins of Carbonado and Framesite». Elements. 1 (2): 85–89. doi:10.2113/gselements.1.2.85.
- ^ Shumilova T, Tkachev S, Isaenko S, Shevchuk S, Rappenglück M, Kazakov V (April 2016). «A «diamond-like star» in the lab. Diamond-like glass». Carbon. 100: 703–709. doi:10.1016/j.carbon.2016.01.068.
- ^ Wei-Haas M. «Life and Rocks May Have Co-Evolved on Earth». Smithsonian. Retrieved September 26, 2017.
- ^ Hesse RW (2007). Jewelrymaking through history. Greenwood Publishing Group. p. 42. ISBN 978-0-313-33507-5.
- ^ Adiga A (April 12, 2004). «Uncommon Brilliance». Time. Archived from the original on March 10, 2007. Retrieved November 3, 2008.
- ^ «Jwaneng». Debswana. Archived from the original on March 17, 2012. Retrieved March 9, 2012.
- ^ a b c Tichotsky J (2000). Russia’s Diamond Colony: The Republic of Sakha. Routledge. p. 254. ISBN 978-90-5702-420-7.
- ^ «Jews Surrender Gem Trade to Indians». Spiegel Online. May 15, 2006.
- ^ «The history of the Antwerp Diamond Center». Antwerp World Diamond Center. 2012-08-16.
- ^ «Commission Decision of 25 July 2001 declaring a concentration to be compatible with the common market and the EEA Agreement». Case No COMP/M.2333 – De Beers/LVMH. EUR-Lex. 2003.
- ^ «Business: Changing facets; Diamonds». The Economist. Vol. 382, no. 8517. 2007. p. 68.
- ^ «Certainty in the Diamond Industry? Watch Out For Tipping Points – IDEX’s Memo». idexonline.com. Retrieved September 24, 2014.
- ^ «The Elusive Sparcle». The Gem & Jewellery Export Promotion Council. Archived from the original on June 16, 2009. Retrieved April 26, 2009.
- ^ Even-Zohar C (November 6, 2008). «Crisis Mitigation at De Beers». DIB online. Archived from the original on May 12, 2011. Retrieved April 26, 2009.
- ^ Even-Zohar C (November 3, 1999). «De Beers to Halve Diamond Stockpile». National Jeweler. Archived from the original on July 5, 2009. Retrieved April 26, 2009.
- ^ «Judgment of the Court of First Instance of 11 July 2007 – Alrosa v Commission». EUR-Lex. 2007. Retrieved April 26, 2009.
- ^ «Mining operations». The De Beers Group. 2007. Archived from the original on June 13, 2008. Retrieved January 4, 2011.
- ^ «Diamond producer Alrosa to resume market diamond sales in May». RIA Novosti. May 6, 2009. Retrieved May 25, 2009.
- ^ «Media releases – Media Centre – Alrosa». Alrosa. December 22, 2009. Archived from the original on August 20, 2013. Retrieved January 4, 2011.
- ^ «Another record profit for BHP». ABC News. August 22, 2007. Retrieved August 23, 2007.
- ^ «Our Companies». Rio Tinto web site. Rio Tinto. Archived from the original on May 11, 2013. Retrieved March 5, 2009.
- ^ «Introduction | IDC». internationaldiamondcouncil.org. Retrieved 2022-10-18.
- ^ a b c Broadman HG, Isik G (2007). Africa’s silk road. World Bank Publications. pp. 297–299. ISBN 978-0-8213-6835-0.
- ^ «Bourse listing». World Federation of Diamond Bourses. Archived from the original on October 25, 2016. Retrieved February 12, 2012.
- ^ «North America Diamond Sales Show No Sign of Slowing». A&W diamonds. Archived from the original on January 6, 2009. Retrieved May 5, 2009.
- ^ a b Pierson HO (1993). Handbook of carbon, graphite, diamond, and fullerenes: properties, processing, and applications. William Andrew. p. 280. ISBN 978-0-8155-1339-1.
- ^ a b James DS (1998). Antique jewellery: its manufacture, materials and design. Osprey Publishing. pp. 82–102. ISBN 978-0-7478-0385-0.
- ^ «The Classical and Special Shapes of Diamonds». kristallsmolensk.com. Retrieved July 14, 2015.
- ^ Prelas MA, Popovici G, Bigelow LK (1998). Handbook of industrial diamonds and diamond films. CRC Press. pp. 984–992. ISBN 978-0-8247-9994-6.
- ^ «Gem Cutting». Popular Mechanics. 74 (5): 760–764. 1940. ISSN 0032-4558.
- ^ Rapaport M. «Keep the Diamond Dream Alive». Rapaport Magazine. Diamonds.net. Retrieved September 9, 2012.
- ^ a b JCK Staff (January 26, 2011). «10 Things Rocking the Industry». JCK. Jckonline.com. Archived from the original on January 7, 2013. Retrieved September 9, 2012.
- ^ a b Epstein EJ (1982). «Have You Ever Tried To Sell a Diamond?». The Atlantic. Retrieved May 5, 2009.
- ^ Bates R (January 14, 2011). «Interview with Forevermark CEO». JCK. Jckonline.com. Archived from the original on November 28, 2012. Retrieved September 9, 2012.
- ^ Harlow GE (1998). The nature of diamonds. Cambridge University Press. p. 34. ISBN 978-0-521-62935-5.
- ^ Kogel JE (2006). Industrial minerals & rocks. Society for Mining, Metallurgy, and Exploration (U.S.). p. 416. ISBN 978-0-87335-233-8.
- ^ «The Australian Diamond Industry». Archived from the original on July 16, 2009. Retrieved August 4, 2009.
- ^ Erlich E, Hausel DW (2002). Diamond deposits: origin, exploration, and history of discovery. SME. p. 158. ISBN 978-0-87335-213-0.
- ^ «Diamond: The mineral Diamond information and pictures». minerals.net. Retrieved September 24, 2014.
- ^ a b c «Industrial Diamonds Statistics and Information». United States Geological Survey. Retrieved May 5, 2009.
- ^ a b Spear KE, Dismukes JP (1994). Synthetic Diamond: Emerging CVD Science and Technology. Wiley–IEEE. p. 628. ISBN 978-0-471-53589-8.
- ^ Holtzapffel C (1856). Turning And Mechanical Manipulation. Holtzapffel & Co. pp. 176–178. ISBN 978-1-879335-39-4.
- ^ Coelho RT, Yamada S, Aspinwall DK, Wise ML (1995). «The application of polycrystalline diamond (PCD) tool materials when drilling and reaming aluminum-based alloys including MMC». International Journal of Machine Tools and Manufacture. 35 (5): 761–774. doi:10.1016/0890-6955(95)93044-7.
- ^ Sakamoto M, Endriz JG, Scifres DR (1992). «120 W CW output power from monolithic AlGaAs (800 nm) laser diode array mounted on diamond heatsink». Electronics Letters. 28 (2): 197–199. Bibcode:1992ElL….28..197S. doi:10.1049/el:19920123.
- ^ a b Yarnell A (2004). «The Many Facets of Man-Made Diamonds». Chemical and Engineering News. 82 (5): 26–31. doi:10.1021/cen-v082n005.p026.
- ^ a b «Conflict Diamonds». United Nations. March 21, 2001. Archived from the original on March 9, 2010. Retrieved May 5, 2009.
- ^ Catelle WR (1911). The Diamond. John Lane Co. p. 159.
- ^ a b c Hershey W (1940). The Book of Diamonds. New York: Hearthside Press. pp. 22–28. ISBN 978-1-4179-7715-4.
- ^ Ball V (1881). «1». Diamonds, Gold and Coal of India. London: Trübner & Co. p. 1. Ball was a geologist in British service.
- ^ «Biggest diamond found in Panna». Mail Today. July 1, 2010. Archived from the original on July 7, 2011.
- ^ Shillington K (2005). Encyclopedia of African history. CRC Press. p. 767. ISBN 978-1-57958-453-5.
- ^ a b Janse AJ (2007). «Global Rough Diamond Production Since 1870». Gems & Gemology. 43 (2): 98–119. doi:10.5741/GEMS.43.2.98.
- ^ a b Lorenz V (2007). «Argyle in Western Australia: The world’s richest diamantiferous pipe; its past and future». Gemmologie, Zeitschrift der Deutschen Gemmologischen Gesellschaft. 56 (1–2): 35–40.
- ^ a b Cooke S (October 17, 2004). «Microscopic diamond found in Montana». The Montana Standard. Archived from the original on January 21, 2005. Retrieved May 5, 2009.
- ^ Marshall S, Shore J (2004). «The Diamond Life». Guerrilla News Network. Archived from the original on January 26, 2007. Retrieved March 21, 2007.
- ^ Shigley JE, Chapman J, Ellison RK (2001). «Discovery and Mining of the Argyle Diamond Deposit, Australia» (PDF). Gems & Gemology. 37 (1): 26–41. doi:10.5741/GEMS.37.1.26. Archived from the original (PDF) on September 30, 2009. Retrieved February 20, 2010.
- ^ a b Basedau M, Mehler A (2005). Resource politics in Sub-Saharan Africa. GIGA-Hamburg. pp. 305–313. ISBN 978-3-928049-91-7.
- ^ World Federation of Diamond Bourses (WFDB) and International Diamond Manufacturers Association: Joint Resolution of 19 July 2000. World Diamond Council. July 19, 2000. ISBN 978-90-04-13656-4. Retrieved November 5, 2006.
- ^ «Voluntary Code of Conduct For Authenticating Canadian Diamond Claims» (PDF). Canadian Diamond Code Committee. 2006. Archived from the original (PDF) on February 29, 2012. Retrieved October 30, 2007.
- ^ Kjarsgaard BA, Levinson AA (2002). «Diamonds in Canada». Gems and Gemology. 38 (3): 208–238. doi:10.5741/GEMS.38.3.208.
- ^ A meta-analysis of the environmental impact specific to diamond mining is in Oluleye G. Environmental Impacts of Mined Diamonds (PDF) (Report). Imperial College London Consultants. Archived (PDF) from the original on 2021-12-03. Retrieved 2022-07-01.
- ^ a b c «The Global Diamond Industry: Lifting the Veil of Mystery» (PDF). Bain & Company. Archived (PDF) from the original on 2012-01-31. Retrieved January 14, 2012.
- ^ Shigley JE, Abbaschian R (2002). «Gemesis Laboratory Created Diamonds». Gems & Gemology. 38 (4): 301–309. doi:10.5741/GEMS.38.4.301.
- ^ Shigley JE, Shen AH, Breeding CM, McClure SF, Shigley JE (2004). «Lab Grown Colored Diamonds from Chatham Created Gems». Gems & Gemology. 40 (2): 128–145. doi:10.5741/GEMS.40.2.128.
- ^ Werner M, Locher R (1998). «Growth and application of undoped and doped diamond films». Reports on Progress in Physics. 61 (12): 1665–1710. Bibcode:1998RPPh…61.1665W. doi:10.1088/0034-4885/61/12/002. S2CID 250878100.
- ^ Pisani B (August 27, 2012). «The Business of Diamonds, From Mining to Retail». CNBC.
- ^ Kogel JE (2006). Industrial Minerals & Rocks. SME. pp. 426–430. ISBN 978-0-87335-233-8.
- ^ O’Donoghue M, Joyner L (2003). Identification of gemstones. Great Britain: Butterworth-Heinemann. pp. 12–19. ISBN 978-0-7506-5512-5.
- ^ Barnard AS (2000). The diamond formula. Butterworth-Heinemann. p. 115. ISBN 978-0-7506-4244-6.
- ^ Shigley JE (2007). «Observations on new coated gemstones». Gemmologie: Zeitschrift der Deutschen Gemmologischen Gesellschaft. 56 (1–2): 53–56.
- ^ US 4488821, Wenckus JF, «Method and means of rapidly distinguishing a simulated diamond from natural diamond», published December 18, 1984, assigned to Ceres Electronics Corporation; U.S. Patent 4,488,821
- ^ a b Edwards HG, Chalmers GM (2005). Raman spectroscopy in archaeology and art history. Royal Society of Chemistry. pp. 387–394. ISBN 978-0-85404-522-8.
- ^ a b Welbourn C (2006). «Identification of Synthetic Diamonds: Present Status and Future Developments, Proceedings of the 4th International Gemological Symposium». Gems and Gemology. 42 (3): 34–35.
- ^ Donahue PJ (April 19, 2004). «DTC Appoints GIA Distributor of DiamondSure and DiamondView». Professional Jeweler Magazine. Retrieved March 2, 2009.
- ^ «SSEF diamond spotter and SSEF illuminator». SSEF Swiss Gemmological Institute. Archived from the original on June 27, 2009. Retrieved May 5, 2009.
- ^ Liddell HG, Scott R. «Adamas». A Greek-English Lexicon. Perseus Project.
- ^ Pliny the Elder (2004). Natural History: A Selection. Penguin Books. p. 371. ISBN 978-0-14-044413-1.
- ^ «Chinese made first use of diamond». BBC News. May 17, 2005. Retrieved March 21, 2007.
- ^ See:
- Lavoisier A (2007-10-15) [1772 (part 2)], «Premier mémoire sur la destruction du diamant par le feu» [First memoir on the destruction of diamond by fire], Histoire de l’Académie royale des sciences, avec les Mémoires de Mathématique & de Physique, tirés des registres de cette Académie [History of the Royal Academy of Sciences, with the Memoirs of Mathematics & Physics, drawn from the records of this academy]] (in French), Gallica: Académie des sciences, pp. 564–591, ISSN 1967-4783, ark:/12148/bpt6k35711, retrieved 2022-07-01
- Lavoisier A (2007-10-15) [1772 (part 2)], «Second mémoire sur la destruction du diamant par le feu» [Second memoir on the destruction of diamond by fire], Histoire de l’Académie royale des sciences, avec les Mémoires de Mathématique & de Physique, tirés des registres de cette Académie (in French), Gallica: Académie des sciences, pp. 591–616, ISSN 1967-4783, ark:/12148/bpt6k35711, retrieved 2022-07-01
- ^ Smithson T (1797) [December 15, 1797]. «On the nature of the diamond». Philosophical Transactions of the Royal Society of London: Giving Some Account of the Present Undertakings, Studies, and Labours of the Ingenious, in Many Considerable Parts of the World. 87: 123–127. doi:10.1098/rstl.1797.0005. S2CID 186213726. Retrieved 2022-07-01.
General and cited references
- Even-Zohar C (2007). From Mine to Mistress: Corporate Strategies and Government Policies in the International Diamond Industry (2nd ed.). Mining Journal Press.
- Davies G (1994). Properties and growth of diamond. INSPEC. ISBN 978-0-85296-875-8.
- O’Donoghue M (2006). Gems. Elsevier. ISBN 978-0-7506-5856-0.
- O’Donoghue M, Joyner L (2003). Identification of gemstones. Great Britain: Butterworth-Heinemann. ISBN 978-0-7506-5512-5.
- Feldman A, Robins LH (1991). Applications of Diamond Films and Related Materials. Elsevier. ISBN 978-1-48329124-6.
- Field JE (1979). The Properties of Diamond. London: Academic Press. ISBN 978-0-12-255350-9.
- Field JE (1992). The Properties of Natural and Synthetic Diamond. London: Academic Press. ISBN 978-0-12-255352-3.
- Hershey W (1940). The Book of Diamonds. Hearthside Press New York. ISBN 978-1-4179-7715-4.
- Koizumi S, Nebel CE, Nesladek M (2008). Physics and Applications of CVD Diamond. Wiley VCH. ISBN 978-3-527-40801-6.
- Pan LS, Kani DR (1995). Diamond: Electronic Properties and Applications. Kluwer Academic Publishers. ISBN 978-0-7923-9524-9.
- Pagel-Theisen V (2001). Diamond Grading ABC: the Manual. Antwerp: Rubin & Son. ISBN 978-3-9800434-6-5.
- Radovic RL, Walker RM, Thrower PA (1965). Chemistry and physics of carbon: a series of advances. New York: Marcel Dekker. ISBN 978-0-8247-0987-7.
- Tolkowsky M (1919). Diamond Design: A Study of the Reflection and Refraction of Light in a Diamond. London: E. & F.N. Spon.
- Wise RW (2016). Secrets of the Gem Trade: The Connoisseur’s Guide to Precious Gemstones (Second ed.). Brunswick House Press. ISBN 978-0-9728223-2-9.
- Zaitsev AM (2001). Optical Properties of Diamond: A Data Handbook. Springer. ISBN 978-3-540-66582-3.
Further reading
- Epstein EJ (February 1982). «Have You Ever Tried to Sell a Diamond?». The Atlantic Monthly. Archived from the original on 15 March 2006. Retrieved 2 January 2023.
- Tyson P (November 2000). «Diamonds in the Sky». «The Diamond Deception». Nova. PBS. Retrieved 2 January 2023.
External links
- Properties of diamond: Ioffe database
- «A Contribution to the Understanding of Blue Fluorescence on the Appearance of Diamonds» at the Wayback Machine (archived 2017-09-08) (2007) Gemological Institute of America (GIA)
Diamond | |
---|---|
![]() The slightly misshapen octahedral shape of this rough diamond crystal in matrix is typical of the mineral. Its lustrous faces also indicate that this crystal is from a primary deposit. | |
General | |
Category | Native minerals |
Formula (repeating unit) | C |
IMA symbol | Dia[1] |
Strunz classification | 1.CB.10a |
Dana classification | 1.3.6.1 |
Crystal system | Cubic |
Crystal class | Hexoctahedral (m3m) H-M symbol: (4/m 3 2/m) |
Space group | Fd3m (No. 227) |
Structure | |
Jmol (3D) | Interactive image |
Identification | |
Formula mass | 12.01 g/mol |
Color | Typically yellow, brown, or gray to colorless. Less often blue, green, black, translucent white, pink, violet, orange, purple, and red. |
Crystal habit | Octahedral |
Twinning | Spinel law common (yielding «macle») |
Cleavage | 111 (perfect in four directions) |
Fracture | Irregular/Uneven |
Mohs scale hardness | 10 (defining mineral) |
Luster | Adamantine |
Streak | Colorless |
Diaphaneity | Transparent to subtransparent to translucent |
Specific gravity | 3.52±0.01 |
Density | 3.5–3.53 g/cm3 3500–3530 kg/m3 |
Polish luster | Adamantine |
Optical properties | Isotropic |
Refractive index | 2.418 (at 500 nm) |
Birefringence | None |
Pleochroism | None |
Dispersion | 0.044 |
Melting point | Pressure dependent |
References | [2][3] |
Main diamond producing countries
Diamond is a solid form of the element carbon with its atoms arranged in a crystal structure called diamond cubic. Another solid form of carbon known as graphite is the chemically stable form of carbon at room temperature and pressure, but diamond is metastable and converts to it at a negligible rate under those conditions. Diamond has the highest hardness and thermal conductivity of any natural material, properties that are used in major industrial applications such as cutting and polishing tools. They are also the reason that diamond anvil cells can subject materials to pressures found deep in the Earth.
Because the arrangement of atoms in diamond is extremely rigid, few types of impurity can contaminate it (two exceptions are boron and nitrogen). Small numbers of defects or impurities (about one per million of lattice atoms) color diamond blue (boron), yellow (nitrogen), brown (defects), green (radiation exposure), purple, pink, orange, or red. Diamond also has a very high refractive index and a relatively high optical dispersion.
Most natural diamonds have ages between 1 billion and 3.5 billion years. Most were formed at depths between 150 and 250 kilometres (93 and 155 mi) in the Earth’s mantle, although a few have come from as deep as 800 kilometres (500 mi). Under high pressure and temperature, carbon-containing fluids dissolved various minerals and replaced them with diamonds. Much more recently (hundreds to tens of million years ago), they were carried to the surface in volcanic eruptions and deposited in igneous rocks known as kimberlites and lamproites.
Synthetic diamonds can be grown from high-purity carbon under high pressures and temperatures or from hydrocarbon gases by chemical vapor deposition (CVD). Imitation diamonds can also be made out of materials such as cubic zirconia and silicon carbide. Natural, synthetic and imitation diamonds are most commonly distinguished using optical techniques or thermal conductivity measurements.
Properties
Diamond is a solid form of pure carbon with its atoms arranged in a crystal. Solid carbon comes in different forms known as allotropes depending on the type of chemical bond. The two most common allotropes of pure carbon are diamond and graphite. In graphite the bonds are sp2 orbital hybrids and the atoms form in planes, with each bound to three nearest neighbors 120 degrees apart. In diamond they are sp3 and the atoms form tetrahedra with each bound to four nearest neighbors.[4][5] Tetrahedra are rigid, the bonds are strong, and of all known substances diamond has the greatest number of atoms per unit volume, which is why it is both the hardest and the least compressible.[6][7] It also has a high density, ranging from 3150 to 3530 kilograms per cubic metre (over three times the density of water) in natural diamonds and 3520 kg/m3 in pure diamond.[2] In graphite, the bonds between nearest neighbors are even stronger, but the bonds between parallel adjacent planes are weak, so the planes easily slip past each other. Thus, graphite is much softer than diamond. However, the stronger bonds make graphite less flammable.[8]
Diamonds have been adopted for many uses because of the material’s exceptional physical characteristics. It has the highest thermal conductivity and the highest sound velocity. It has low adhesion and friction, and its coefficient of thermal expansion is extremely low. Its optical transparency extends from the far infrared to the deep ultraviolet and it has high optical dispersion. It also has high electrical resistance. It is chemically inert, not reacting with most corrosive substances, and has excellent biological compatibility.[9]
Thermodynamics
The equilibrium pressure and temperature conditions for a transition between graphite and diamond are well established theoretically and experimentally. The equilibrium pressure varies linearly with temperature, between 1.7 GPa at 0 K and 12 GPa at 5000 K (the diamond/graphite/liquid triple point).[10][11]
However, the phases have a wide region about this line where they can coexist. At normal temperature and pressure, 20 °C (293 K) and 1 standard atmosphere (0.10 MPa), the stable phase of carbon is graphite, but diamond is metastable and its rate of conversion to graphite is negligible.[7] However, at temperatures above about 4500 K, diamond rapidly converts to graphite. Rapid conversion of graphite to diamond requires pressures well above the equilibrium line: at 2000 K, a pressure of 35 GPa is needed.[10]
Above the graphite-diamond-liquid carbon triple point, the melting point of diamond increases slowly with increasing pressure; but at pressures of hundreds of GPa, it decreases.[12] At high pressures, silicon and germanium have a BC8 body-centered cubic crystal structure, and a similar structure is predicted for carbon at high pressures. At 0 K, the transition is predicted to occur at 1100 GPa.[13]
Research results published in an article in the scientific journal Nature Physics in 2010 suggest that at ultrahigh pressures and temperatures (about 10 million atmospheres or 1 TPa and 50,000 °C) diamond melts into a metallic fluid. The extreme conditions required for this to occur are present in the ice giants Neptune and Uranus. Both planets are made up of approximately 10 percent carbon and could hypothetically contain oceans of liquid carbon. Since large quantities of metallic fluid can affect the magnetic field, this could serve as an explanation as to why the geographic and magnetic poles of the two planets are unaligned.[14][15]
Crystal structure
Diamond unit cell, showing the tetrahedral structure
The most common crystal structure of diamond is called diamond cubic. It is formed of unit cells (see the figure) stacked together. Although there are 18 atoms in the figure, each corner atom is shared by eight unit cells and each atom in the center of a face is shared by two, so there are a total of eight atoms per unit cell.[16] The length of each side of the unit cell is denoted by a and is 3.567 angstroms.[17]
The nearest neighbour distance in the diamond lattice is 1.732a/4 where a is the lattice constant, usually given in Angstrøms as a = 3.567 Å, which is 0.3567 nm.
A diamond cubic lattice can be thought of as two interpenetrating face-centered cubic lattices with one displaced by 1⁄4 of the diagonal along a cubic cell, or as one lattice with two atoms associated with each lattice point.[17] Viewed from a <1 1 1> crystallographic direction, it is formed of layers stacked in a repeating ABCABC … pattern. Diamonds can also form an ABAB … structure, which is known as hexagonal diamond or lonsdaleite, but this is far less common and is formed under different conditions from cubic carbon.[18]
Crystal habit
One face of an uncut octahedral diamond, showing trigons (of positive and negative relief) formed by natural chemical etching
Diamonds occur most often as euhedral or rounded octahedra and twinned octahedra known as macles. As diamond’s crystal structure has a cubic arrangement of the atoms, they have many facets that belong to a cube, octahedron, rhombicosidodecahedron, tetrakis hexahedron, or disdyakis dodecahedron. The crystals can have rounded-off and unexpressive edges and can be elongated. Diamonds (especially those with rounded crystal faces) are commonly found coated in nyf, an opaque gum-like skin.[19]
Some diamonds contain opaque fibers. They are referred to as opaque if the fibers grow from a clear substrate or fibrous if they occupy the entire crystal. Their colors range from yellow to green or gray, sometimes with cloud-like white to gray impurities. Their most common shape is cuboidal, but they can also form octahedra, dodecahedra, macles, or combined shapes. The structure is the result of numerous impurities with sizes between 1 and 5 microns. These diamonds probably formed in kimberlite magma and sampled the volatiles.[20]
Diamonds can also form polycrystalline aggregates. There have been attempts to classify them into groups with names such as boart, ballas, stewartite, and framesite, but there is no widely accepted set of criteria.[20] Carbonado, a type in which the diamond grains were sintered (fused without melting by the application of heat and pressure), is black in color and tougher than single crystal diamond.[21] It has never been observed in a volcanic rock. There are many theories for its origin, including formation in a star, but no consensus.[20][22][23]
Mechanical
Hardness
The extreme hardness of diamond in certain orientations makes it useful in materials science, as in this pyramidal diamond embedded in the working surface of a Vickers hardness tester.
Diamond is the hardest known natural material on both the Vickers scale and the Mohs scale. Diamond’s great hardness relative to other materials has been known since antiquity, and is the source of its name. This does not mean that it is infinitely hard, indestructible, or unscratchable.[24] Indeed, diamonds can be scratched by other diamonds[25] and worn down over time even by softer materials, such as vinyl phonograph records.[26]
Diamond hardness depends on its purity, crystalline perfection, and orientation: hardness is higher for flawless, pure crystals oriented to the <111> direction (along the longest diagonal of the cubic diamond lattice).[27] Therefore, whereas it might be possible to scratch some diamonds with other materials, such as boron nitride, the hardest diamonds can only be scratched by other diamonds and nanocrystalline diamond aggregates.
The hardness of diamond contributes to its suitability as a gemstone. Because it can only be scratched by other diamonds, it maintains its polish extremely well. Unlike many other gems, it is well-suited to daily wear because of its resistance to scratching—perhaps contributing to its popularity as the preferred gem in engagement or wedding rings, which are often worn every day.
The hardest natural diamonds mostly originate from the Copeton and Bingara fields located in the New England area in New South Wales, Australia. These diamonds are generally small, perfect to semiperfect octahedra, and are used to polish other diamonds. Their hardness is associated with the crystal growth form, which is single-stage crystal growth. Most other diamonds show more evidence of multiple growth stages, which produce inclusions, flaws, and defect planes in the crystal lattice, all of which affect their hardness. It is possible to treat regular diamonds under a combination of high pressure and high temperature to produce diamonds that are harder than the diamonds used in hardness gauges.[28]
Diamonds cut glass, but this does not positively identify a diamond because other materials, such as quartz, also lie above glass on the Mohs scale and can also cut it. Diamonds can scratch other diamonds, but this can result in damage to one or both stones. Hardness tests are infrequently used in practical gemology because of their potentially destructive nature.[29] The extreme hardness and high value of diamond means that gems are typically polished slowly, using painstaking traditional techniques and greater attention to detail than is the case with most other gemstones;[30] these tend to result in extremely flat, highly polished facets with exceptionally sharp facet edges. Diamonds also possess an extremely high refractive index and fairly high dispersion. Taken together, these factors affect the overall appearance of a polished diamond and most diamantaires still rely upon skilled use of a loupe (magnifying glass) to identify diamonds «by eye».[31]
Toughness
Somewhat related to hardness is another mechanical property toughness, which is a material’s ability to resist breakage from forceful impact. The toughness of natural diamond has been measured as 7.5–10 MPa·m1/2.[32][33] This value is good compared to other ceramic materials, but poor compared to most engineering materials such as engineering alloys, which typically exhibit toughnesses over 100 MPa·m1/2. As with any material, the macroscopic geometry of a diamond contributes to its resistance to breakage. Diamond has a cleavage plane and is therefore more fragile in some orientations than others. Diamond cutters use this attribute to cleave some stones, prior to faceting.[34] «Impact toughness» is one of the main indexes to measure the quality of synthetic industrial diamonds.
Yield strength
Diamond has compressive yield strength of 130–140 GPa.[35] This exceptionally high value, along with the hardness and transparency of diamond, are the reasons that diamond anvil cells are the main tool for high pressure experiments.[36] These anvils have reached pressures of 600 GPa.[37] Much higher pressures may be possible with nanocrystalline diamonds.[36][37]
Elasticity and tensile strength
Usually, attempting to deform bulk diamond crystal by tension or bending results in brittle fracture. However, when single crystalline diamond is in the form of micro/nanoscale wires or needles (~100–300 nanometers in diameter, micrometers long), they can be elastically stretched by as much as 9-10 percent tensile strain without failure,[38] with a maximum local tensile stress of ~89 to 98 GPa,[39] very close to the theoretical limit for this material.[40]
Electrical conductivity
Other specialized applications also exist or are being developed, including use as semiconductors: some blue diamonds are natural semiconductors, in contrast to most diamonds, which are excellent electrical insulators. The conductivity and blue color originate from boron impurity. Boron substitutes for carbon atoms in the diamond lattice, donating a hole into the valence band.[41]
Substantial conductivity is commonly observed in nominally undoped diamond grown by chemical vapor deposition. This conductivity is associated with hydrogen-related species adsorbed at the surface, and it can be removed by annealing or other surface treatments.[42][43]
Thin needles of diamond can be made to vary their electronic band gap from the normal 5.6 eV to near zero by selective mechanical deformation.[44]
High-purity diamond wafers 5 cm in diameter exhibit perfect resistance in one direction and perfect conductance in the other, creating the possibility of using them for quantum data storage. The material contains only 3 parts per million of nitrogen. The diamond was grown on a stepped substrate, which eliminated cracking.[45]
Surface property
Diamonds are naturally lipophilic and hydrophobic, which means the diamonds’ surface cannot be wet by water, but can be easily wet and stuck by oil. This property can be utilized to extract diamonds using oil when making synthetic diamonds. However, when diamond surfaces are chemically modified with certain ions, they are expected to become so hydrophilic that they can stabilize multiple layers of water ice at human body temperature.[46]
The surface of diamonds is partially oxidized. The oxidized surface can be reduced by heat treatment under hydrogen flow. That is to say, this heat treatment partially removes oxygen-containing functional groups. But diamonds (sp3C) are unstable against high temperature (above about 400 °C (752 °F)) under atmospheric pressure. The structure gradually changes into sp2C above this temperature. Thus, diamonds should be reduced under this temperature.[47]
Chemical stability
At room temperature, diamonds do not react with any chemical reagents including strong acids and bases.
In an atmosphere of pure oxygen, diamond has an ignition point that ranges from 690 °C (1,274 °F) to 840 °C (1,540 °F); smaller crystals tend to burn more easily. It increases in temperature from red to white heat and burns with a pale blue flame, and continues to burn after the source of heat is removed. By contrast, in air the combustion will cease as soon as the heat is removed because the oxygen is diluted with nitrogen. A clear, flawless, transparent diamond is completely converted to carbon dioxide; any impurities will be left as ash.[48] Heat generated from cutting a diamond will not ignite the diamond,[49] and neither will a cigarette lighter,[50] but house fires and blow torches are hot enough. Jewelers must be careful when molding the metal in a diamond ring.[51]
Diamond powder of an appropriate grain size (around 50 microns) burns with a shower of sparks after ignition from a flame. Consequently, pyrotechnic compositions based on synthetic diamond powder can be prepared. The resulting sparks are of the usual red-orange color, comparable to charcoal, but show a very linear trajectory which is explained by their high density.[52] Diamond also reacts with fluorine gas above about 700 °C (1,292 °F).
Color
Diamond has a wide band gap of 5.5 eV corresponding to the deep ultraviolet wavelength of 225 nanometers. This means that pure diamond should transmit visible light and appear as a clear colorless crystal. Colors in diamond originate from lattice defects and impurities. The diamond crystal lattice is exceptionally strong, and only atoms of nitrogen, boron, and hydrogen can be introduced into diamond during the growth at significant concentrations (up to atomic percents). Transition metals nickel and cobalt, which are commonly used for growth of synthetic diamond by high-pressure high-temperature techniques, have been detected in diamond as individual atoms; the maximum concentration is 0.01% for nickel[53] and even less for cobalt. Virtually any element can be introduced to diamond by ion implantation.[54]
Nitrogen is by far the most common impurity found in gem diamonds and is responsible for the yellow and brown color in diamonds. Boron is responsible for the blue color.[55] Color in diamond has two additional sources: irradiation (usually by alpha particles), that causes the color in green diamonds, and plastic deformation of the diamond crystal lattice. Plastic deformation is the cause of color in some brown[56] and perhaps pink and red diamonds.[57] In order of increasing rarity, yellow diamond is followed by brown, colorless, then by blue, green, black, pink, orange, purple, and red.[34] «Black», or carbonado, diamonds are not truly black, but rather contain numerous dark inclusions that give the gems their dark appearance. Colored diamonds contain impurities or structural defects that cause the coloration, while pure or nearly pure diamonds are transparent and colorless. Most diamond impurities replace a carbon atom in the crystal lattice, known as a carbon flaw. The most common impurity, nitrogen, causes a slight to intense yellow coloration depending upon the type and concentration of nitrogen present.[34] The Gemological Institute of America (GIA) classifies low saturation yellow and brown diamonds as diamonds in the normal color range, and applies a grading scale from «D» (colorless) to «Z» (light yellow). Yellow diamonds of high color saturation or a different color, such as pink or blue, are called fancy colored diamonds and fall under a different grading scale.[34]
In 2008, the Wittelsbach Diamond, a 35.56-carat (7.112 g) blue diamond once belonging to the King of Spain, fetched over US$24 million at a Christie’s auction.[58] In May 2009, a 7.03-carat (1.406 g) blue diamond fetched the highest price per carat ever paid for a diamond when it was sold at auction for 10.5 million Swiss francs (6.97 million euros, or US$9.5 million at the time).[59] That record was, however, beaten the same year: a 5-carat (1.0 g) vivid pink diamond was sold for $10.8 million in Hong Kong on December 1, 2009.[60]
Clarity
Clarity is one of the 4C’s (color, clarity, cut and carat weight) that helps in identifying the quality of diamonds. The Gemological Institute of America (GIA) developed 11 clarity scales to decide the quality of a diamond for its sale value. The GIA clarity scale spans from Flawless (FL) to included (I) having internally flawless (IF), very, very slightly included (VVS), very slightly included (VS) and slightly included (SI) in between. Impurities in natural diamonds are due to the presence of natural minerals and oxides. The clarity scale grades the diamond based on the color, size, location of impurity and quantity of clarity visible under 10x magnification.[61] Inclusions in diamond can be extracted by optical methods. The process is to take pre-enhancement images, identifying the inclusion removal part and finally removing the diamond facets and noises.[62]
Fluorescence
Extremely rare purple fluorescent diamonds from the Ellendale L-Channel deposit in Australia
Between 25% to 35% of natural diamonds exhibit some degree of fluorescence when examined under invisible long-wave Ultraviolet light or higher energy radiation sources such as X-rays and lasers.[63] Incandescent lighting will not cause a diamond to fluoresce. Diamonds can fluoresce in a variety of colours including blue (most common), orange, yellow, white, green and very rarely red and purple. Although the causes are not well understood, variations in the atomic structure, such as the number of nitrogen atoms present are thought to contribute to the phenomenon.
Thermal Conductivity
Diamonds can be identified by their high thermal conductivity (900–2320 W·m−1·K−1).[64] Their high refractive index is also indicative, but other materials have similar refractivity.
Geology
Diamonds are extremely rare, with concentrations of at most parts per billion in source rock.[20] Before the 20th century, most diamonds were found in alluvial deposits. Loose diamonds are also found along existing and ancient shorelines, where they tend to accumulate because of their size and density.[65]: 149 Rarely, they have been found in glacial till (notably in Wisconsin and Indiana), but these deposits are not of commercial quality.[65]: 19 These types of deposit were derived from localized igneous intrusions through weathering and transport by wind or water.[66]
Most diamonds come from the Earth’s mantle, and most of this section discusses those diamonds. However, there are other sources. Some blocks of the crust, or terranes, have been buried deep enough as the crust thickened so they experienced ultra-high-pressure metamorphism. These have evenly distributed microdiamonds that show no sign of transport by magma. In addition, when meteorites strike the ground, the shock wave can produce high enough temperatures and pressures for microdiamonds and nanodiamonds to form.[66] Impact-type microdiamonds can be used as an indicator of ancient impact craters.[67] Popigai impact structure in Russia may have the world’s largest diamond deposit, estimated at trillions of carats, and formed by an asteroid impact.[68]
A common misconception is that diamonds form from highly compressed coal. Coal is formed from buried prehistoric plants, and most diamonds that have been dated are far older than the first land plants. It is possible that diamonds can form from coal in subduction zones, but diamonds formed in this way are rare, and the carbon source is more likely carbonate rocks and organic carbon in sediments, rather than coal.[69][70]
Surface distribution
Diamonds are far from evenly distributed over the Earth. A rule of thumb known as Clifford’s rule states that they are almost always found in kimberlites on the oldest part of cratons, the stable cores of continents with typical ages of 2.5 billion years or more.[66][71]: 314 However, there are exceptions. The Argyle diamond mine in Australia, the largest producer of diamonds by weight in the world, is located in a mobile belt, also known as an orogenic belt,[72] a weaker zone surrounding the central craton that has undergone compressional tectonics. Instead of kimberlite, the host rock is lamproite. Lamproites with diamonds that are not economically viable are also found in the United States, India, and Australia.[66] In addition, diamonds in the Wawa belt of the Superior province in Canada and microdiamonds in the island arc of Japan are found in a type of rock called lamprophyre.[66]
Kimberlites can be found in narrow (1 to 4 meters) dikes and sills, and in pipes with diameters that range from about 75 m to 1.5 km. Fresh rock is dark bluish green to greenish gray, but after exposure rapidly turns brown and crumbles.[73] It is hybrid rock with a chaotic mixture of small minerals and rock fragments (clasts) up to the size of watermelons. They are a mixture of xenocrysts and xenoliths (minerals and rocks carried up from the lower crust and mantle), pieces of surface rock, altered minerals such as serpentine, and new minerals that crystallized during the eruption. The texture varies with depth. The composition forms a continuum with carbonatites, but the latter have too much oxygen for carbon to exist in a pure form. Instead, it is locked up in the mineral calcite (CaCO
3).[66]
All three of the diamond-bearing rocks (kimberlite, lamproite and lamprophyre) lack certain minerals (melilite and kalsilite) that are incompatible with diamond formation. In kimberlite, olivine is large and conspicuous, while lamproite has Ti-phlogopite and lamprophyre has biotite and amphibole. They are all derived from magma types that erupt rapidly from small amounts of melt, are rich in volatiles and magnesium oxide, and are less oxidizing than more common mantle melts such as basalt. These characteristics allow the melts to carry diamonds to the surface before they dissolve.[66]
Exploration
Diavik Mine, on an island in Lac de Gras in northern Canada
Kimberlite pipes can be difficult to find. They weather quickly (within a few years after exposure) and tend to have lower topographic relief than surrounding rock. If they are visible in outcrops, the diamonds are never visible because they are so rare. In any case, kimberlites are often covered with vegetation, sediments, soils, or lakes. In modern searches, geophysical methods such as aeromagnetic surveys, electrical resistivity, and gravimetry, help identify promising regions to explore. This is aided by isotopic dating and modeling of the geological history. Then surveyors must go to the area and collect samples, looking for kimberlite fragments or indicator minerals. The latter have compositions that reflect the conditions where diamonds form, such as extreme melt depletion or high pressures in eclogites. However, indicator minerals can be misleading; a better approach is geothermobarometry, where the compositions of minerals are analyzed as if they were in equilibrium with mantle minerals.[66]
Finding kimberlites requires persistence, and only a small fraction contain diamonds that are commercially viable. The only major discoveries since about 1980 have been in Canada. Since existing mines have lifetimes of as little as 25 years, there could be a shortage of new diamonds in the future.[66]
Ages
Diamonds are dated by analyzing inclusions using the decay of radioactive isotopes. Depending on the elemental abundances, one can look at the decay of rubidium to strontium, samarium to neodymium, uranium to lead, argon-40 to argon-39, or rhenium to osmium. Those found in kimberlites have ages ranging from 1 to 3.5 billion years, and there can be multiple ages in the same kimberlite, indicating multiple episodes of diamond formation. The kimberlites themselves are much younger. Most of them have ages between tens of millions and 300 million years old, although there are some older exceptions (Argyle, Premier and Wawa). Thus, the kimberlites formed independently of the diamonds and served only to transport them to the surface.[20][66] Kimberlites are also much younger than the cratons they have erupted through. The reason for the lack of older kimberlites is unknown, but it suggests there was some change in mantle chemistry or tectonics. No kimberlite has erupted in human history.[66]
Origin in mantle
Red garnet inclusion in a diamond[74]
Most gem-quality diamonds come from depths of 150–250 km in the lithosphere. Such depths occur below cratons in mantle keels, the thickest part of the lithosphere. These regions have high enough pressure and temperature to allow diamonds to form and they are not convecting, so diamonds can be stored for billions of years until a kimberlite eruption samples them.[66]
Host rocks in a mantle keel include harzburgite and lherzolite, two type of peridotite. The most dominant rock type in the upper mantle, peridotite is an igneous rock consisting mostly of the minerals olivine and pyroxene; it is low in silica and high in magnesium. However, diamonds in peridotite rarely survive the trip to the surface.[66] Another common source that does keep diamonds intact is eclogite, a metamorphic rock that typically forms from basalt as an oceanic plate plunges into the mantle at a subduction zone.[20]
A smaller fraction of diamonds (about 150 have been studied) come from depths of 330–660 km, a region that includes the transition zone. They formed in eclogite but are distinguished from diamonds of shallower origin by inclusions of majorite (a form of garnet with excess silicon). A similar proportion of diamonds comes from the lower mantle at depths between 660 and 800 km.[20]
Diamond is thermodynamically stable at high pressures and temperatures, with the phase transition from graphite occurring at greater temperatures as the pressure increases. Thus, underneath continents it becomes stable at temperatures of 950 degrees Celsius and pressures of 4.5 gigapascals, corresponding to depths of 150 kilometers or greater. In subduction zones, which are colder, it becomes stable at temperatures of 800 °C and pressures of 3.5 gigapascals. At depths greater than 240 km, iron-nickel metal phases are present and carbon is likely to be either dissolved in them or in the form of carbides. Thus, the deeper origin of some diamonds may reflect unusual growth environments.[20][66]
In 2018 the first known natural samples of a phase of ice called Ice VII were found as inclusions in diamond samples. The inclusions formed at depths between 400 and 800 km, straddling the upper and lower mantle, and provide evidence for water-rich fluid at these depths.[75][76]
Carbon sources
The mantle has roughly one billion gigatonnes of carbon (for comparison, the atmosphere-ocean system has about 44,000 gigatonnes).[77] Carbon has two stable isotopes, 12C and 13C, in a ratio of approximately 99:1 by mass.[66] This ratio has a wide range in meteorites, which implies that it also varied a lot in the early Earth. It can also be altered by surface processes like photosynthesis. The fraction is generally compared to a standard sample using a ratio δ13C expressed in parts per thousand. Common rocks from the mantle such as basalts, carbonatites, and kimberlites have ratios between −8 and −2. On the surface, organic sediments have an average of −25 while carbonates have an average of 0.[20]
Populations of diamonds from different sources have distributions of δ13C that vary markedly. Peridotitic diamonds are mostly within the typical mantle range; eclogitic diamonds have values from −40 to +3, although the peak of the distribution is in the mantle range. This variability implies that they are not formed from carbon that is primordial (having resided in the mantle since the Earth formed). Instead, they are the result of tectonic processes, although (given the ages of diamonds) not necessarily the same tectonic processes that act in the present.[66]
Formation and growth
Age zones in a diamond.[74]
Diamonds in the mantle form through a metasomatic process where a C-O-H-N-S fluid or melt dissolves minerals in a rock and replaces them with new minerals. (The vague term C-O-H-N-S is commonly used because the exact composition is not known.) Diamonds form from this fluid either by reduction of oxidized carbon (e.g., CO2 or CO3) or oxidation of a reduced phase such as methane.[20]
Using probes such as polarized light, photoluminescence, and cathodoluminescence, a series of growth zones can be identified in diamonds. The characteristic pattern in diamonds from the lithosphere involves a nearly concentric series of zones with very thin oscillations in luminescence and alternating episodes where the carbon is resorbed by the fluid and then grown again. Diamonds from below the lithosphere have a more irregular, almost polycrystalline texture, reflecting the higher temperatures and pressures as well as the transport of the diamonds by convection.[66]
Transport to the surface
Diagram of a volcanic pipe
Geological evidence supports a model in which kimberlite magma rises at 4–20 meters per second, creating an upward path by hydraulic fracturing of the rock. As the pressure decreases, a vapor phase exsolves from the magma, and this helps to keep the magma fluid. At the surface, the initial eruption explodes out through fissures at high speeds (over 200 m/s (450 mph)). Then, at lower pressures, the rock is eroded, forming a pipe and producing fragmented rock (breccia). As the eruption wanes, there is pyroclastic phase and then metamorphism and hydration produces serpentinites.[66]
Double diamonds
Double diamond discovered in the Ellendale Diamond Field, Western Australia
In rare cases, diamonds have been found that contain a cavity within which is a second diamond. The first double diamond, the Matryoshka, was found by Alrosa in Yakutia, Russia, in 2019.[78] Another one was found in the Ellendale Diamond Field in Western Australia in 2021.[79]
In space
Although diamonds on Earth are rare, they are very common in space. In meteorites, about three percent of the carbon is in the form of nanodiamonds, having diameters of a few nanometers. Sufficiently small diamonds can form in the cold of space because their lower surface energy makes them more stable than graphite. The isotopic signatures of some nanodiamonds indicate they were formed outside the Solar System in stars.[80]
High pressure experiments predict that large quantities of diamonds condense from methane into a «diamond rain» on the ice giant planets Uranus and Neptune.[81][82][83] Some extrasolar planets may be almost entirely composed of diamond.[84]
Diamonds may exist in carbon-rich stars, particularly white dwarfs. One theory for the origin of carbonado, the toughest form of diamond, is that it originated in a white dwarf or supernova.[85][86] Diamonds formed in stars may have been the first minerals.[87]
Industry
The most familiar uses of diamonds today are as gemstones used for adornment, and as industrial abrasives for cutting hard materials. The markets for gem-grade and industrial-grade diamonds value diamonds differently.
Gem-grade diamonds
The dispersion of white light into spectral colors is the primary gemological characteristic of gem diamonds. In the 20th century, experts in gemology developed methods of grading diamonds and other gemstones based on the characteristics most important to their value as a gem. Four characteristics, known informally as the four Cs, are now commonly used as the basic descriptors of diamonds: these are its mass in carats (a carat being equal to 0.2 grams), cut (quality of the cut is graded according to proportions, symmetry and polish), color (how close to white or colorless; for fancy diamonds how intense is its hue), and clarity (how free is it from inclusions). A large, flawless diamond is known as a paragon.[88]
A large trade in gem-grade diamonds exists. Although most gem-grade diamonds are sold newly polished, there is a well-established market for resale of polished diamonds (e.g. pawnbroking, auctions, second-hand jewelry stores, diamantaires, bourses, etc.). One hallmark of the trade in gem-quality diamonds is its remarkable concentration: wholesale trade and diamond cutting is limited to just a few locations; in 2003, 92% of the world’s diamonds were cut and polished in Surat, India.[89] Other important centers of diamond cutting and trading are the Antwerp diamond district in Belgium, where the International Gemological Institute is based, London, the Diamond District in New York City, the Diamond Exchange District in Tel Aviv and Amsterdam. One contributory factor is the geological nature of diamond deposits: several large primary kimberlite-pipe mines each account for significant portions of market share (such as the Jwaneng mine in Botswana, which is a single large-pit mine that can produce between 12,500,000 and 15,000,000 carats (2,500 and 3,000 kg) of diamonds per year[90]). Secondary alluvial diamond deposits, on the other hand, tend to be fragmented amongst many different operators because they can be dispersed over many hundreds of square kilometers (e.g., alluvial deposits in Brazil).[citation needed]
The production and distribution of diamonds is largely consolidated in the hands of a few key players, and concentrated in traditional diamond trading centers, the most important being Antwerp, where 80% of all rough diamonds, 50% of all cut diamonds and more than 50% of all rough, cut and industrial diamonds combined are handled.[91] This makes Antwerp a de facto «world diamond capital».[92] The city of Antwerp also hosts the Antwerpsche Diamantkring, created in 1929 to become the first and biggest diamond bourse dedicated to rough diamonds.[93] Another important diamond center is New York City, where almost 80% of the world’s diamonds are sold, including auction sales.[91]
The De Beers company, as the world’s largest diamond mining company, holds a dominant position in the industry, and has done so since soon after its founding in 1888 by the British businessman Cecil Rhodes. De Beers is currently the world’s largest operator of diamond production facilities (mines) and distribution channels for gem-quality diamonds. The Diamond Trading Company (DTC) is a subsidiary of De Beers and markets rough diamonds from De Beers-operated mines. De Beers and its subsidiaries own mines that produce some 40% of annual world diamond production. For most of the 20th century over 80% of the world’s rough diamonds passed through De Beers,[94] but by 2001–2009 the figure had decreased to around 45%,[95] and by 2013 the company’s market share had further decreased to around 38% in value terms and even less by volume.[96] De Beers sold off the vast majority of its diamond stockpile in the late 1990s – early 2000s[97] and the remainder largely represents working stock (diamonds that are being sorted before sale).[98] This was well documented in the press[99] but remains little known to the general public.
As a part of reducing its influence, De Beers withdrew from purchasing diamonds on the open market in 1999 and ceased, at the end of 2008, purchasing Russian diamonds mined by the largest Russian diamond company Alrosa.[100] As of January 2011, De Beers states that it only sells diamonds from the following four countries: Botswana, Namibia, South Africa and Canada.[101] Alrosa had to suspend their sales in October 2008 due to the global energy crisis,[102] but the company reported that it had resumed selling rough diamonds on the open market by October 2009.[103] Apart from Alrosa, other important diamond mining companies include BHP, which is the world’s largest mining company;[104] Rio Tinto, the owner of the Argyle (100%), Diavik (60%), and Murowa (78%) diamond mines;[105] and Petra Diamonds, the owner of several major diamond mines in Africa.
Diamond polisher in Amsterdam
Further down the supply chain, members of The World Federation of Diamond Bourses (WFDB) act as a medium for wholesale diamond exchange, trading both polished and rough diamonds. The WFDB consists of independent diamond bourses in major cutting centers such as Tel Aviv, Antwerp, Johannesburg and other cities across the US, Europe and Asia.[34] In 2000, the WFDB and The International Diamond Manufacturers Association established the World Diamond Council to prevent the trading of diamonds used to fund war and inhumane acts. WFDB’s additional activities include sponsoring the World Diamond Congress every two years, as well as the establishment of the International Diamond Council (IDC) to oversee diamond grading.[106]
Once purchased by Sightholders (which is a trademark term referring to the companies that have a three-year supply contract with DTC), diamonds are cut and polished in preparation for sale as gemstones (‘industrial’ stones are regarded as a by-product of the gemstone market; they are used for abrasives).[107] The cutting and polishing of rough diamonds is a specialized skill that is concentrated in a limited number of locations worldwide.[107] Traditional diamond cutting centers are Antwerp, Amsterdam, Johannesburg, New York City, and Tel Aviv. Recently, diamond cutting centers have been established in China, India, Thailand, Namibia and Botswana.[107] Cutting centers with lower cost of labor, notably Surat in Gujarat, India, handle a larger number of smaller carat diamonds, while smaller quantities of larger or more valuable diamonds are more likely to be handled in Europe or North America. The recent expansion of this industry in India, employing low cost labor, has allowed smaller diamonds to be prepared as gems in greater quantities than was previously economically feasible.[91]
Diamonds prepared as gemstones are sold on diamond exchanges called bourses. There are 28 registered diamond bourses in the world.[108] Bourses are the final tightly controlled step in the diamond supply chain; wholesalers and even retailers are able to buy relatively small lots of diamonds at the bourses, after which they are prepared for final sale to the consumer. Diamonds can be sold already set in jewelry, or sold unset («loose»). According to the Rio Tinto, in 2002 the diamonds produced and released to the market were valued at US$9 billion as rough diamonds, US$14 billion after being cut and polished, US$28 billion in wholesale diamond jewelry, and US$57 billion in retail sales.[109]
Cutting
The Daria-i-Noor Diamond—an example of unusual diamond cut and jewelry arrangement.
Mined rough diamonds are converted into gems through a multi-step process called «cutting». Diamonds are extremely hard, but also brittle and can be split up by a single blow. Therefore, diamond cutting is traditionally considered as a delicate procedure requiring skills, scientific knowledge, tools and experience. Its final goal is to produce a faceted jewel where the specific angles between the facets would optimize the diamond luster, that is dispersion of white light, whereas the number and area of facets would determine the weight of the final product. The weight reduction upon cutting is significant and can be of the order of 50%.[110] Several possible shapes are considered, but the final decision is often determined not only by scientific, but also practical considerations. For example, the diamond might be intended for display or for wear, in a ring or a necklace, singled or surrounded by other gems of certain color and shape.[111] Some of them may be considered as classical, such as round, pear, marquise, oval, hearts and arrows diamonds, etc. Some of them are special, produced by certain companies, for example, Phoenix, Cushion, Sole Mio diamonds, etc.[112]
The most time-consuming part of the cutting is the preliminary analysis of the rough stone. It needs to address a large number of issues, bears much responsibility, and therefore can last years in case of unique diamonds. The following issues are considered:
- The hardness of diamond and its ability to cleave strongly depend on the crystal orientation. Therefore, the crystallographic structure of the diamond to be cut is analyzed using X-ray diffraction to choose the optimal cutting directions.
- Most diamonds contain visible non-diamond inclusions and crystal flaws. The cutter has to decide which flaws are to be removed by the cutting and which could be kept.
- The diamond can be split by a single, well calculated blow of a hammer to a pointed tool, which is quick, but risky. Alternatively, it can be cut with a diamond saw, which is a more reliable but tedious procedure.[111][113]
After initial cutting, the diamond is shaped in numerous stages of polishing. Unlike cutting, which is a responsible but quick operation, polishing removes material by gradual erosion and is extremely time-consuming. The associated technique is well developed; it is considered as a routine and can be performed by technicians.[114] After polishing, the diamond is reexamined for possible flaws, either remaining or induced by the process. Those flaws are concealed through various diamond enhancement techniques, such as repolishing, crack filling, or clever arrangement of the stone in the jewelry. Remaining non-diamond inclusions are removed through laser drilling and filling of the voids produced.[29]
Marketing
Diamond Balance Scale 0.01 — 25 Carat Jewelers Measuring Tool
Marketing has significantly affected the image of diamond as a valuable commodity.
N. W. Ayer & Son, the advertising firm retained by De Beers in the mid-20th century, succeeded in reviving the American diamond market and the firm created new markets in countries where no diamond tradition had existed before. N. W. Ayer’s marketing included product placement, advertising focused on the diamond product itself rather than the De Beers brand, and associations with celebrities and royalty. Without advertising the De Beers brand, De Beers was advertising its competitors’ diamond products as well,[115] but this was not a concern as De Beers dominated the diamond market throughout the 20th century. De Beers’ market share dipped temporarily to second place in the global market below Alrosa in the aftermath of the global economic crisis of 2008, down to less than 29% in terms of carats mined, rather than sold.[116] The campaign lasted for decades but was effectively discontinued by early 2011. De Beers still advertises diamonds, but the advertising now mostly promotes its own brands, or licensed product lines, rather than completely «generic» diamond products.[116] The campaign was perhaps best captured by the slogan «a diamond is forever».[117] This slogan is now being used by De Beers Diamond Jewelers,[118] a jewelry firm which is a 50/50% joint venture between the De Beers mining company and LVMH, the luxury goods conglomerate.
Brown-colored diamonds constituted a significant part of the diamond production, and were predominantly used for industrial purposes. They were seen as worthless for jewelry (not even being assessed on the diamond color scale). After the development of Argyle diamond mine in Australia in 1986, and marketing, brown diamonds have become acceptable gems.[119][120] The change was mostly due to the numbers: the Argyle mine, with its 35,000,000 carats (7,000 kg) of diamonds per year, makes about one-third of global production of natural diamonds;[121] 80% of Argyle diamonds are brown.[122]
Industrial-grade diamonds
A scalpel with synthetic diamond blade
Close-up photograph of an angle grinder blade with tiny diamonds shown embedded in the metal
A diamond knife blade used for cutting ultrathin sections (typically 70 to 350 nm) for transmission electron microscopy
Industrial diamonds are valued mostly for their hardness and thermal conductivity, making many of the gemological characteristics of diamonds, such as the 4 Cs, irrelevant for most applications. Eighty percent of mined diamonds (equal to about 135,000,000 carats (27,000 kg) annually) are unsuitable for use as gemstones and are used industrially.[123] In addition to mined diamonds, synthetic diamonds found industrial applications almost immediately after their invention in the 1950s; in 2014, 4,500,000,000 carats (900,000 kg) of synthetic diamonds were produced, 90% of which were produced in China. Approximately 90% of diamond grinding grit is currently of synthetic origin.[124]
The boundary between gem-quality diamonds and industrial diamonds is poorly defined and partly depends on market conditions (for example, if demand for polished diamonds is high, some lower-grade stones will be polished into low-quality or small gemstones rather than being sold for industrial use). Within the category of industrial diamonds, there is a sub-category comprising the lowest-quality, mostly opaque stones, which are known as bort.[125]
Industrial use of diamonds has historically been associated with their hardness, which makes diamond the ideal material for cutting and grinding tools. As the hardest known naturally occurring material, diamond can be used to polish, cut, or wear away any material, including other diamonds. Common industrial applications of this property include diamond-tipped drill bits and saws, and the use of diamond powder as an abrasive. Less expensive industrial-grade diamonds (bort) with more flaws and poorer color than gems, are used for such purposes.[126] Diamond is not suitable for machining ferrous alloys at high speeds, as carbon is soluble in iron at the high temperatures created by high-speed machining, leading to greatly increased wear on diamond tools compared to alternatives.[127]
Specialized applications include use in laboratories as containment for high-pressure experiments (see diamond anvil cell), high-performance bearings, and limited use in specialized windows.[125] With the continuing advances being made in the production of synthetic diamonds, future applications are becoming feasible. The high thermal conductivity of diamond makes it suitable as a heat sink for integrated circuits in electronics.[128]
Mining
Approximately 130,000,000 carats (26,000 kg) of diamonds are mined annually, with a total value of nearly US$9 billion, and about 100,000 kg (220,000 lb) are synthesized annually.[129]
Roughly 49% of diamonds originate from Central and Southern Africa, although significant sources of the mineral have been discovered in Canada, India, Russia, Brazil, and Australia.[124] They are mined from kimberlite and lamproite volcanic pipes, which can bring diamond crystals, originating from deep within the Earth where high pressures and temperatures enable them to form, to the surface. The mining and distribution of natural diamonds are subjects of frequent controversy such as concerns over the sale of blood diamonds or conflict diamonds by African paramilitary groups.[130] The diamond supply chain is controlled by a limited number of powerful businesses, and is also highly concentrated in a small number of locations around the world.
Only a very small fraction of the diamond ore consists of actual diamonds. The ore is crushed, during which care is required not to destroy larger diamonds, and then sorted by density. Today, diamonds are located in the diamond-rich density fraction with the help of X-ray fluorescence, after which the final sorting steps are done by hand. Before the use of X-rays became commonplace,[110] the separation was done with grease belts; diamonds have a stronger tendency to stick to grease than the other minerals in the ore.[34]
Historically, diamonds were found only in alluvial deposits in Guntur and Krishna district of the Krishna River delta in Southern India.[131] India led the world in diamond production from the time of their discovery in approximately the 9th century BC[132][133] to the mid-18th century AD, but the commercial potential of these sources had been exhausted by the late 18th century and at that time India was eclipsed by Brazil where the first non-Indian diamonds were found in 1725.[132] Currently, one of the most prominent Indian mines is located at Panna.[134]
Diamond extraction from primary deposits (kimberlites and lamproites) started in the 1870s after the discovery of the Diamond Fields in South Africa.[135] Production has increased over time and now an accumulated total of 4,500,000,000 carats (900,000 kg) have been mined since that date.[136] Twenty percent of that amount has been mined in the last five years, and during the last 10 years, nine new mines have started production; four more are waiting to be opened soon. Most of these mines are located in Canada, Zimbabwe, Angola, and one in Russia.[136]
In the U.S., diamonds have been found in Arkansas, Colorado, New Mexico, Wyoming, and Montana.[137][138] In 2004, the discovery of a microscopic diamond in the U.S. led to the January 2008 bulk-sampling of kimberlite pipes in a remote part of Montana. The Crater of Diamonds State Park in Arkansas is open to the public, and is the only mine in the world where members of the public can dig for diamonds.[138]
Today, most commercially viable diamond deposits are in Russia (mostly in Sakha Republic, for example Mir pipe and Udachnaya pipe), Botswana, Australia (Northern and Western Australia) and the Democratic Republic of the Congo.[139] In 2005, Russia produced almost one-fifth of the global diamond output, according to the British Geological Survey. Australia boasts the richest diamantiferous pipe, with production from the Argyle diamond mine reaching peak levels of 42 metric tons per year in the 1990s.[137][140] There are also commercial deposits being actively mined in the Northwest Territories of Canada and Brazil.[124] Diamond prospectors continue to search the globe for diamond-bearing kimberlite and lamproite pipes.
Political issues
In some of the more politically unstable central African and west African countries, revolutionary groups have taken control of diamond mines, using proceeds from diamond sales to finance their operations. Diamonds sold through this process are known as conflict diamonds or blood diamonds.[130]
In response to public concerns that their diamond purchases were contributing to war and human rights abuses in central and western Africa, the United Nations, the diamond industry and diamond-trading nations introduced the Kimberley Process in 2002.[141] The Kimberley Process aims to ensure that conflict diamonds do not become intermixed with the diamonds not controlled by such rebel groups. This is done by requiring diamond-producing countries to provide proof that the money they make from selling the diamonds is not used to fund criminal or revolutionary activities. Although the Kimberley Process has been moderately successful in limiting the number of conflict diamonds entering the market, some still find their way in. According to the International Diamond Manufacturers Association, conflict diamonds constitute 2–3% of all diamonds traded.[142] Two major flaws still hinder the effectiveness of the Kimberley Process: (1) the relative ease of smuggling diamonds across African borders, and (2) the violent nature of diamond mining in nations that are not in a technical state of war and whose diamonds are therefore considered «clean».[141]
The Canadian Government has set up a body known as the Canadian Diamond Code of Conduct[143] to help authenticate Canadian diamonds. This is a stringent tracking system of diamonds and helps protect the «conflict free» label of Canadian diamonds.[144]
Mineral resource exploitation in general causes irreversible environmental damage, which must be weighed against the socio-economic benefits to a country.[145]
Synthetics, simulants, and enhancements
Synthetics
Synthetic diamonds are diamonds manufactured in a laboratory, as opposed to diamonds mined from the Earth. The gemological and industrial uses of diamond have created a large demand for rough stones. This demand has been satisfied in large part by synthetic diamonds, which have been manufactured by various processes for more than half a century. However, in recent years it has become possible to produce gem-quality synthetic diamonds of significant size.[65] It is possible to make colorless synthetic gemstones that, on a molecular level, are identical to natural stones and so visually similar that only a gemologist with special equipment can tell the difference.[146]
The majority of commercially available synthetic diamonds are yellow and are produced by so-called high-pressure high-temperature (HPHT) processes.[147] The yellow color is caused by nitrogen impurities. Other colors may also be reproduced such as blue, green or pink, which are a result of the addition of boron or from irradiation after synthesis.[148]
Another popular method of growing synthetic diamond is chemical vapor deposition (CVD). The growth occurs under low pressure (below atmospheric pressure). It involves feeding a mixture of gases (typically 1 to 99 methane to hydrogen) into a chamber and splitting them into chemically active radicals in a plasma ignited by microwaves, hot filament, arc discharge, welding torch, or laser.[149] This method is mostly used for coatings, but can also produce single crystals several millimeters in size (see picture).[129]
As of 2010, nearly all 5,000 million carats (1,000 tonnes) of synthetic diamonds produced per year are for industrial use. Around 50% of the 133 million carats of natural diamonds mined per year end up in industrial use.[146][150] Mining companies’ expenses average 40 to 60 US dollars per carat for natural colorless diamonds, while synthetic manufacturers’ expenses average $2,500 per carat for synthetic, gem-quality colorless diamonds.[146]: 79 However, a purchaser is more likely to encounter a synthetic when looking for a fancy-colored diamond because nearly all synthetic diamonds are fancy-colored, while only 0.01% of natural diamonds are.[151]
-
Synthetic diamonds of various colors grown by the high-pressure high-temperature technique
-
Colorless gem cut from diamond grown by chemical vapor deposition
Simulants
Gem-cut synthetic silicon carbide set in a ring
A diamond simulant is a non-diamond material that is used to simulate the appearance of a diamond, and may be referred to as diamante. Cubic zirconia is the most common. The gemstone moissanite (silicon carbide) can be treated as a diamond simulant, though more costly to produce than cubic zirconia. Both are produced synthetically.[152]
Enhancements
Diamond enhancements are specific treatments performed on natural or synthetic diamonds (usually those already cut and polished into a gem), which are designed to better the gemological characteristics of the stone in one or more ways. These include laser drilling to remove inclusions, application of sealants to fill cracks, treatments to improve a white diamond’s color grade, and treatments to give fancy color to a white diamond.[153]
Coatings are increasingly used to give a diamond simulant such as cubic zirconia a more «diamond-like» appearance. One such substance is diamond-like carbon—an amorphous carbonaceous material that has some physical properties similar to those of the diamond. Advertising suggests that such a coating would transfer some of these diamond-like properties to the coated stone, hence enhancing the diamond simulant. Techniques such as Raman spectroscopy should easily identify such a treatment.[154]
Identification
Early diamond identification tests included a scratch test relying on the superior hardness of diamond. This test is destructive, as a diamond can scratch another diamond, and is rarely used nowadays. Instead, diamond identification relies on its superior thermal conductivity. Electronic thermal probes are widely used in the gemological centers to separate diamonds from their imitations. These probes consist of a pair of battery-powered thermistors mounted in a fine copper tip. One thermistor functions as a heating device while the other measures the temperature of the copper tip: if the stone being tested is a diamond, it will conduct the tip’s thermal energy rapidly enough to produce a measurable temperature drop. This test takes about two to three seconds.[155]
Whereas the thermal probe can separate diamonds from most of their simulants, distinguishing between various types of diamond, for example synthetic or natural, irradiated or non-irradiated, etc., requires more advanced, optical techniques. Those techniques are also used for some diamonds simulants, such as silicon carbide, which pass the thermal conductivity test. Optical techniques can distinguish between natural diamonds and synthetic diamonds. They can also identify the vast majority of treated natural diamonds.[156] «Perfect» crystals (at the atomic lattice level) have never been found, so both natural and synthetic diamonds always possess characteristic imperfections, arising from the circumstances of their crystal growth, that allow them to be distinguished from each other.[157]
Laboratories use techniques such as spectroscopy, microscopy, and luminescence under shortwave ultraviolet light to determine a diamond’s origin.[156] They also use specially made instruments to aid them in the identification process. Two screening instruments are the DiamondSure and the DiamondView, both produced by the DTC and marketed by the GIA.[158]
Several methods for identifying synthetic diamonds can be performed, depending on the method of production and the color of the diamond. CVD diamonds can usually be identified by an orange fluorescence. D-J colored diamonds can be screened through the Swiss Gemmological Institute’s[159] Diamond Spotter. Stones in the D-Z color range can be examined through the DiamondSure UV/visible spectrometer, a tool developed by De Beers.[157] Similarly, natural diamonds usually have minor imperfections and flaws, such as inclusions of foreign material, that are not seen in synthetic diamonds.
Screening devices based on diamond type detection can be used to make a distinction between diamonds that are certainly natural and diamonds that are potentially synthetic. Those potentially synthetic diamonds require more investigation in a specialized lab. Examples of commercial screening devices are D-Screen (WTOCD / HRD Antwerp), Alpha Diamond Analyzer (Bruker / HRD Antwerp), and D-Secure (DRC Techno).
Etymology, earliest use and composition discovery
The name diamond is derived from Ancient Greek: ἀδάμας (adámas), ‘proper, unalterable, unbreakable, untamed’, from ἀ- (a-), ‘not’ + Ancient Greek: δαμάω (damáō), ‘to overpower, tame’.[160] Diamonds are thought to have been first recognized and mined in India, where significant alluvial deposits of the stone could be found many centuries ago along the rivers Penner, Krishna, and Godavari. Diamonds have been known in India for at least 3,000 years but most likely 6,000 years.[132]
Diamonds have been treasured as gemstones since their use as religious icons in ancient India. Their usage in engraving tools also dates to early human history.[161][162] The popularity of diamonds has risen since the 19th century because of increased supply, improved cutting and polishing techniques, growth in the world economy, and innovative and successful advertising campaigns.[117]
In 1772, the French scientist Antoine Lavoisier used a lens to concentrate the rays of the sun on a diamond in an atmosphere of oxygen, and showed that the only product of the combustion was carbon dioxide, proving that diamond is composed of carbon.[163] Later in 1797, the English chemist Smithson Tennant repeated and expanded that experiment.[164] By demonstrating that burning diamond and graphite releases the same amount of gas, he established the chemical equivalence of these substances.[30]
See also
- Deep carbon cycle
- Diamondoid
- List of diamonds
- List of largest rough diamonds
- List of minerals
- Superhard material
Citations
- ^ Warr LN (2021). «IMA–CNMNC approved mineral symbols». Mineralogical Magazine. 85 (3): 291–320. Bibcode:2021MinM…85..291W. doi:10.1180/mgm.2021.43. S2CID 235729616.
- ^ a b «Diamond». Mindat. Retrieved July 7, 2009.
- ^ «Diamond». WebMineral. Retrieved July 7, 2009.
- ^ Delhaes P (2000). «Polymorphism of carbon». In Delhaes P (ed.). Graphite and precursors. Gordon & Breach. pp. 1–24. ISBN 978-90-5699-228-6.
- ^ Pierson HO (2012). Handbook of carbon, graphite, diamond, and fullerenes: properties, processing, and applications. Noyes Publications. pp. 40–41. ISBN 978-0-8155-1739-9.
- ^ Angus JC (1997). «Structure and thermochemistry of diamond». In Paoletti A, Tucciarone A (eds.). The physics of diamond. IOS Press. pp. 9–30. ISBN 978-1-61499-220-2.
- ^ a b Rock PA (1983). Chemical Thermodynamics. University Science Books. pp. 257–260. ISBN 978-1-891389-32-0.
- ^ Gray T (October 8, 2009). «Gone in a Flash». Popular Science. Retrieved October 31, 2018.
- ^ Chen Y, Zhang L (2013). Polishing of diamond materials: mechanisms, modeling and implementation. Springer Science & Business Media. pp. 1–2. ISBN 978-1-84996-408-1.
- ^ a b Bundy P, Bassett WA, Weathers MS, Hemley RJ, Mao HK, Goncharov AF (1996). «The pressure-temperature phase and transformation diagram for carbon; updated through 1994». Carbon. 34 (2): 141–153. doi:10.1016/0008-6223(96)00170-4.
- ^ Wang CX, Yang GW (2012). «Thermodynamic and kinetic approaches of diamond and related nanomaterials formed by laser ablation in liquid». In Yang G (ed.). Laser ablation in liquids: principles and applications in the preparation of nanomaterials. Pan Stanford Pub. pp. 164–165. ISBN 978-981-4241-52-6.
- ^ Wang X, Scandolo S, Car R (October 2005). «Carbon phase diagram from ab initio molecular dynamics». Physical Review Letters. 95 (18): 185701. Bibcode:2005PhRvL..95r5701W. doi:10.1103/PhysRevLett.95.185701. PMID 16383918.
- ^ Correa AA, Bonev SA, Galli G (January 2006). «Carbon under extreme conditions: phase boundaries and electronic properties from first-principles theory». Proceedings of the National Academy of Sciences of the United States of America. 103 (5): 1204–1208. Bibcode:2006PNAS..103.1204C. doi:10.1073/pnas.0510489103. PMC 1345714. PMID 16432191.
- ^ Bland E (January 15, 2010). «Diamond oceans possible on Uranus, Neptune». Discovery News. Archived from the original on 2012-03-11. Retrieved January 16, 2010.
- ^ Silvera I (2010). «Diamond: Molten under pressure». Nature Physics. 6 (1): 9–10. Bibcode:2010NatPh…6….9S. doi:10.1038/nphys1491. S2CID 120836330.
- ^ Rajendran V (2004). Materials science. Tata McGraw-Hill Pub. p. 2.16. ISBN 978-0-07-058369-6.
- ^ a b Ashcroft NW, Mermin ND (1976). Solid state physics. Holt, Rinehart and Winston. p. 76. ISBN 978-0-03-083993-1.
- ^ Bandosz TJ, Biggs MJ, Gubbins KE, Hattori Y, Iiyama T, Kaneko T, Pikunic J, Thomson K (2003). «Molecular models of porous carbons». In Radovic LR (ed.). Chemistry and physics of carbon. Vol. 28. Marcel Dekker. pp. 46–47. ISBN 978-0-8247-0987-7.
- ^ Webster R, Read PG (2000). Gems: Their sources, descriptions and identification (5th ed.). Great Britain: Butterworth-Heinemann. p. 17. ISBN 978-0-7506-1674-4.
- ^ a b c d e f g h i j Cartigny P, Palot M, Thomassot E, Harris JW (May 30, 2014). «Diamond Formation: A Stable Isotope Perspective». Annual Review of Earth and Planetary Sciences. 42 (1): 699–732. Bibcode:2014AREPS..42..699C. doi:10.1146/annurev-earth-042711-105259.
- ^ Fukura S, Nakagawa T, Kagi H (November 2005). «High spatial resolution photoluminescence and Raman spectroscopic measurements of a natural polycrystalline diamond, carbonado». Diamond and Related Materials. 14 (11–12): 1950–1954. Bibcode:2005DRM….14.1950F. doi:10.1016/j.diamond.2005.08.046.
- ^ Mohammad G, Siddiquei MM, Abu El-Asrar AM (2006). «Poly (ADP-ribose) polymerase mediates diabetes-induced retinal neuropathy». Mediators of Inflammation. 2013 (2): 510451. arXiv:physics/0608014. Bibcode:2006ApJ…653L.153G. doi:10.1086/510451. PMC 3857786. PMID 24347828. S2CID 59405368.
- ^ «Diamonds from Outer Space: Geologists Discover Origin of Earth’s Mysterious Black Diamonds». National Science Foundation. January 8, 2007. Retrieved October 28, 2007.
- ^ «Diamonds Are Indestructible, Right?». Dominion Jewelers. 2015-12-16. Retrieved 2020-10-31.
- ^ M. Seal, «The abrasion of diamond», Proceedings of the Royal Society A 248:1254 (25 November 1958) doi:10.1098/rspa.1958.0250
- ^ Weiler HD (2021-04-13) [1954], The wear and care of records and styli, retrieved 2022-01-01 – via Shure
- ^ Neves AJ, Nazaré MH (2001). Properties, Growth and Applications of Diamond. Institution of Engineering and Technology. pp. 142–147. ISBN 978-0-85296-785-0.
- ^ Boser U (2008). «Diamonds on Demand». Smithsonian. 39 (3): 52–59.
- ^ a b Read PG (2005). Gemmology. Butterworth-Heinemann. pp. 165–166. ISBN 978-0-7506-6449-3.
- ^ a b Hazen RM (1999). The diamond makers. Cambridge University Press. pp. 7–10. ISBN 978-0-521-65474-6.
- ^ O’Donoghue M (1997). Synthetic, Imitation and Treated Gemstones. Gulf Professional Publishing. pp. 34–37. ISBN 978-0-7506-3173-0.
- ^ Lee J, Novikov NV (2005). Innovative superhard materials and sustainable coatings for advanced manufacturing. Springer. p. 102. ISBN 978-0-8493-3512-9.
- ^ Marinescu ID, Tönshoff HK, Inasaki I (2000). Handbook of ceramic grinding and polishing. William Andrew. p. 21. ISBN 978-0-8155-1424-4.
- ^ a b c d e f Harlow GE (1998). The nature of diamonds. Cambridge University Press. pp. 223, 230–249. ISBN 978-0-521-62935-5.
- ^ Eremets MI, Trojan IA, Gwaze P, Huth J, Boehler R, Blank VD (October 3, 2005). «The strength of diamond». Applied Physics Letters. 87 (14): 141902. Bibcode:2005ApPhL..87n1902E. doi:10.1063/1.2061853.
- ^ a b Dubrovinsky L, Dubrovinskaia N, Prakapenka VB, Abakumov AM (October 23, 2012). «Implementation of micro-ball nanodiamond anvils for high-pressure studies above 6 Mbar». Nature Communications. 3 (1): 1163. Bibcode:2012NatCo…3.1163D. doi:10.1038/ncomms2160. PMC 3493652. PMID 23093199.
- ^ a b Wogan T (November 2, 2012). «Improved diamond anvil cell allows higher pressures than ever before». Physics World. Nature Communications. Retrieved 2022-07-01.
- ^ Dang C, Chou JP, Dai B, Chou CT, Yang Y, Fan R, et al. (January 2021). «Achieving large uniform tensile elasticity in microfabricated diamond». Science. 371 (6524): 76–78. Bibcode:2021Sci…371…76D. doi:10.1126/science.abc4174. PMID 33384375.
- ^ Banerjee A, Bernoulli D, Zhang H, Yuen MF, Liu J, Dong J, et al. (April 2018). «Ultralarge elastic deformation of nanoscale diamond». Science. 360 (6386): 300–302. Bibcode:2018Sci…360..300B. doi:10.1126/science.aar4165. PMID 29674589.
- ^ LLorca J (April 2018). «On the quest for the strongest materials». Science. 360 (6386): 264–265. arXiv:2105.05099. Bibcode:2018Sci…360..264L. doi:10.1126/science.aat5211. PMID 29674578. S2CID 4986592.
- ^ Collins AT (1993). «The Optical and Electronic Properties of Semiconducting Diamond». Philosophical Transactions of the Royal Society A. 342 (1664): 233–244. Bibcode:1993RSPTA.342..233C. doi:10.1098/rsta.1993.0017. S2CID 202574625.
- ^ Landstrass MI, Ravi KV (1989). «Resistivity of chemical vapor deposited diamond films». Applied Physics Letters. 55 (10): 975–977. Bibcode:1989ApPhL..55..975L. doi:10.1063/1.101694.
- ^ Zhang W, Ristein J, Ley L (October 2008). «Hydrogen-terminated diamond electrodes. II. Redox activity». Physical Review. E, Statistical, Nonlinear, and Soft Matter Physics. 78 (4 Pt 1): 041603. Bibcode:2008PhRvE..78d1603Z. doi:10.1103/PhysRevE.78.041603. PMID 18999435.
- ^ Shi Z, Dao M, Tsymbalov E, Shapeev A, Li J, Suresh S (October 2020). «Metallization of diamond». Proceedings of the National Academy of Sciences of the United States of America. 117 (40): 24634–24639. Bibcode:2020PNAS..11724634S. doi:10.1073/pnas.2013565117. PMC 7547227. PMID 33020306.
- ^ Irving M (2022-04-28). «Two-inch diamond wafers could store a billion Blu-Ray’s worth of data». New Atlas. Retrieved 2022-04-29.
- ^ Wissner-Gross AD, Kaxiras E (August 2007). «Diamond stabilization of ice multilayers at human body temperature» (PDF). Physical Review. E, Statistical, Nonlinear, and Soft Matter Physics. 76 (2 Pt 1): 020501. Bibcode:2007PhRvE..76b0501W. doi:10.1103/physreve.76.020501. PMID 17929997. S2CID 44344503. Archived (PDF) from the original on 2011-07-24.
- ^ Fujimoto A, Yamada Y, Koinuma M, Sato S (June 2016). «Origins of sp(3)C peaks in C1s X-ray Photoelectron Spectra of Carbon Materials». Analytical Chemistry. 88 (12): 6110–6114. doi:10.1021/acs.analchem.6b01327. PMID 27264720.
- ^ Bauer M (2012). Precious Stones. Vol. 1. Dover Publications. pp. 115–117. ISBN 978-0-486-15125-0.
- ^ «Diamond Care and Cleaning Guide». Gemological Institute of America. Retrieved 1 August 2019.
- ^ Jones C (27 August 2016). «Diamonds are Flammable! How to Safeguard Your Jewelry». DMIA. Retrieved 1 August 2019.
- ^ Baird CS. «Can you light diamond on fire?». Science Questions with Surprising Answers. Retrieved 1 August 2019.
- ^ Lederle F, Koch J, Hübner EG (February 21, 2019). «Colored Sparks». European Journal of Inorganic Chemistry. 2019 (7): 928–937. doi:10.1002/ejic.201801300. S2CID 104449284.
- ^ Collins AT, Kanda H, Isoya J, Ammerlaan CA, Van Wyk JA (1998). «Correlation between optical absorption and EPR in high-pressure diamond grown from a nickel solvent catalyst». Diamond and Related Materials. 7 (2–5): 333–338. Bibcode:1998DRM…..7..333C. doi:10.1016/S0925-9635(97)00270-7.
- ^ Zaitsev AM (2000). «Vibronic spectra of impurity-related optical centers in diamond». Physical Review B. 61 (19): 12909–12922. Bibcode:2000PhRvB..6112909Z. doi:10.1103/PhysRevB.61.12909.
- ^ Walker J (1979). «Optical absorption and luminescence in diamond» (PDF). Reports on Progress in Physics. 42 (10): 1605–1659. Bibcode:1979RPPh…42.1605W. CiteSeerX 10.1.1.467.443. doi:10.1088/0034-4885/42/10/001. S2CID 250857323. Archived (PDF) from the original on 2015-09-06.
- ^ Hounsome LS, Jones R, Shaw MJ, Briddon PR, Öberg S, Briddon P, Öberg S (2006). «Origin of brown coloration in diamond». Physical Review B. 73 (12): 125203. Bibcode:2006PhRvB..73l5203H. doi:10.1103/PhysRevB.73.125203.
- ^ Wise RW (2001). Secrets Of The Gem Trade, The Connoisseur’s Guide To Precious Gemstones. Brunswick House Press. pp. 223–224. ISBN 978-0-9728223-8-1.
- ^ Khan U (December 10, 2008). «Blue-grey diamond belonging to King of Spain has sold for record 16.3 GBP». The Daily Telegraph. London. Retrieved March 31, 2010.
- ^
Nebehay S (May 12, 2009). «Rare blue diamond sells for record $9.5 million». Reuters. Retrieved May 13, 2009. - ^ Pomfret J (December 1, 2009). «Vivid pink diamond sells for record $10.8 million». Reuters.
- ^ Cowing MD (2014). «Objective ciamond clarity grading» (PDF). Journal of Gemmology. 34 (4): 316–332. doi:10.15506/JoG.2014.34.4.316. Archived (PDF) from the original on 2021-04-18. Retrieved 19 September 2021.
- ^ Wang W, Cai L (September 2019). «Inclusion extraction from diamond clarity images based on the analysis of diamond optical properties». Optics Express. 27 (19): 27242–27255. Bibcode:2019OExpr..2727242W. doi:10.1364/OE.27.027242. PMID 31674589. S2CID 203141270.
- ^ «Fact Checking Diamond Fluorescence: 11 Myths Dispelled». GIA 4Cs. 2018-03-27. Retrieved 2022-06-06.
- ^ Wei L, Kuo PK, Thomas RL, Anthony TR, Banholzer WF (June 1993). «Thermal conductivity of isotopically modified single crystal diamond». Physical Review Letters. 70 (24): 3764–3767. Bibcode:1993PhRvL..70.3764W. doi:10.1103/PhysRevLett.70.3764. PMID 10053956.
- ^ a b c Erlich EI, Hausel WD (2002). Diamond deposits: origin, exploration, and history of discovery. Littleton, CO: Society for Mining, Metallurgy, and Exploration. ISBN 978-0-87335-213-0.
- ^ a b c d e f g h i j k l m n o p q r Shirey SB, Shigley JE (December 1, 2013). «Recent Advances in Understanding the Geology of Diamonds». Gems & Gemology. 49 (4): 188–222. doi:10.5741/GEMS.49.4.188.
- ^ Carlson RW (2005). The Mantle and Core. Elsevier. p. 248. ISBN 978-0-08-044848-0.
- ^ Deutsch A, Masaitis VL, Langenhorst F, Grieve RA (2000). «Popigai, Siberia—well preserved giant impact structure, national treasury, and world’s geological heritage». Episodes. 23 (1): 3–12. doi:10.18814/epiiugs/2000/v23i1/002.
- ^ King H (2012). «How do diamonds form? They don’t form from coal!». Geology and Earth Science News and Information. geology.com. Archived from the original on October 30, 2013. Retrieved June 29, 2012.
- ^ Pak-Harvey A (October 31, 2013). «10 common scientific misconceptions». The Christian Science Monitor. Retrieved August 30, 2017.
- ^ Pohl WL (2011). Economic Geology: Principles and Practice. John Wiley & Sons. ISBN 978-1-4443-9486-3.
- ^ Allaby M (2013). «mobile belt». A dictionary of geology and earth sciences (4th ed.). Oxford: Oxford University Press. ISBN 978-0-19-174433-4.
- ^ Kjarsgaard BA (2007). «Kimberlite pipe models: significance for exploration» (PDF). In Milkereit B (ed.). Proceedings of Exploration 07: Fifth Decennial International Conference on Mineral Exploration. Decennial Mineral Exploration Conferences, 2007. pp. 667–677. Archived (PDF) from the original on 2012-12-24. Retrieved March 1, 2018.
- ^ a b Deep Carbon Observatory (2019). Deep Carbon Observatory: A Decade of Discovery. Washington, DC. doi:10.17863/CAM.44064. Archived from the original on 17 December 2019. Retrieved 13 December 2019.
- ^ Cartier K (April 2, 2018). «Diamond Impurities Reveal Water Deep Within the Mantle». Eos. 99. doi:10.1029/2018EO095949.
- ^ Perkins S (March 8, 2018). «Pockets of water may lie deep below Earth’s surface». Science.
- ^ Lee CA, Jiang H, Dasgupta R, Torres M (2019). «A Framework for Understanding Whole-Earth Carbon Cycling». In Orcutt BN, Daniel I, Dasgupta R (eds.). Deep carbon: past to present. Cambridge University Press. pp. 313–357. doi:10.1017/9781108677950.011. ISBN 978-1-108-67795-0. S2CID 210787128.
- ^ Wei-Haas M (2019-10-10). «Bizarre ‘nesting doll’ diamond found inside another diamond». National Geographic. Retrieved 2021-11-27.
- ^ Fowler C (2021-11-26). «Rare ‘double diamond’ discovery comes as race to restart mothballed Ellendale mine heats up». Australian Broadcasting Corporation. Retrieved 2021-11-27.
- ^ Tielens AG (July 12, 2013). «The molecular universe». Reviews of Modern Physics. 85 (3): 1021–1081. Bibcode:2013RvMP…85.1021T. doi:10.1103/RevModPhys.85.1021.
- ^ Kerr RA (October 1999). «Neptune may crush methane into diamonds». Science. 286 (5437): 25. doi:10.1126/science.286.5437.25a. PMID 10532884. S2CID 42814647.
- ^ Scandolo S, Jeanloz R (November–December 2003). «The Centers of Planets: In laboratories and computers, shocked and squeezed matter turns metallic, coughs up diamonds and reveals Earth’s white-hot center». American Scientist. 91 (6): 516–525. Bibcode:2003AmSci..91..516S. doi:10.1511/2003.38.905. JSTOR 27858301. S2CID 120975663.
- ^ Kaplan S (August 25, 2017). «It rains solid diamonds on Uranus and Neptune». The Washington Post. Retrieved October 16, 2017.
- ^ Max Planck Institute for Radio Astronomy (August 25, 2011). «A planet made of diamond». Astronomy magazine. Retrieved September 25, 2017.
- ^ Heaney PJ, de Vicenzi EP (2005). «Strange Diamonds: the Mysterious Origins of Carbonado and Framesite». Elements. 1 (2): 85–89. doi:10.2113/gselements.1.2.85.
- ^ Shumilova T, Tkachev S, Isaenko S, Shevchuk S, Rappenglück M, Kazakov V (April 2016). «A «diamond-like star» in the lab. Diamond-like glass». Carbon. 100: 703–709. doi:10.1016/j.carbon.2016.01.068.
- ^ Wei-Haas M. «Life and Rocks May Have Co-Evolved on Earth». Smithsonian. Retrieved September 26, 2017.
- ^ Hesse RW (2007). Jewelrymaking through history. Greenwood Publishing Group. p. 42. ISBN 978-0-313-33507-5.
- ^ Adiga A (April 12, 2004). «Uncommon Brilliance». Time. Archived from the original on March 10, 2007. Retrieved November 3, 2008.
- ^ «Jwaneng». Debswana. Archived from the original on March 17, 2012. Retrieved March 9, 2012.
- ^ a b c Tichotsky J (2000). Russia’s Diamond Colony: The Republic of Sakha. Routledge. p. 254. ISBN 978-90-5702-420-7.
- ^ «Jews Surrender Gem Trade to Indians». Spiegel Online. May 15, 2006.
- ^ «The history of the Antwerp Diamond Center». Antwerp World Diamond Center. 2012-08-16.
- ^ «Commission Decision of 25 July 2001 declaring a concentration to be compatible with the common market and the EEA Agreement». Case No COMP/M.2333 – De Beers/LVMH. EUR-Lex. 2003.
- ^ «Business: Changing facets; Diamonds». The Economist. Vol. 382, no. 8517. 2007. p. 68.
- ^ «Certainty in the Diamond Industry? Watch Out For Tipping Points – IDEX’s Memo». idexonline.com. Retrieved September 24, 2014.
- ^ «The Elusive Sparcle». The Gem & Jewellery Export Promotion Council. Archived from the original on June 16, 2009. Retrieved April 26, 2009.
- ^ Even-Zohar C (November 6, 2008). «Crisis Mitigation at De Beers». DIB online. Archived from the original on May 12, 2011. Retrieved April 26, 2009.
- ^ Even-Zohar C (November 3, 1999). «De Beers to Halve Diamond Stockpile». National Jeweler. Archived from the original on July 5, 2009. Retrieved April 26, 2009.
- ^ «Judgment of the Court of First Instance of 11 July 2007 – Alrosa v Commission». EUR-Lex. 2007. Retrieved April 26, 2009.
- ^ «Mining operations». The De Beers Group. 2007. Archived from the original on June 13, 2008. Retrieved January 4, 2011.
- ^ «Diamond producer Alrosa to resume market diamond sales in May». RIA Novosti. May 6, 2009. Retrieved May 25, 2009.
- ^ «Media releases – Media Centre – Alrosa». Alrosa. December 22, 2009. Archived from the original on August 20, 2013. Retrieved January 4, 2011.
- ^ «Another record profit for BHP». ABC News. August 22, 2007. Retrieved August 23, 2007.
- ^ «Our Companies». Rio Tinto web site. Rio Tinto. Archived from the original on May 11, 2013. Retrieved March 5, 2009.
- ^ «Introduction | IDC». internationaldiamondcouncil.org. Retrieved 2022-10-18.
- ^ a b c Broadman HG, Isik G (2007). Africa’s silk road. World Bank Publications. pp. 297–299. ISBN 978-0-8213-6835-0.
- ^ «Bourse listing». World Federation of Diamond Bourses. Archived from the original on October 25, 2016. Retrieved February 12, 2012.
- ^ «North America Diamond Sales Show No Sign of Slowing». A&W diamonds. Archived from the original on January 6, 2009. Retrieved May 5, 2009.
- ^ a b Pierson HO (1993). Handbook of carbon, graphite, diamond, and fullerenes: properties, processing, and applications. William Andrew. p. 280. ISBN 978-0-8155-1339-1.
- ^ a b James DS (1998). Antique jewellery: its manufacture, materials and design. Osprey Publishing. pp. 82–102. ISBN 978-0-7478-0385-0.
- ^ «The Classical and Special Shapes of Diamonds». kristallsmolensk.com. Retrieved July 14, 2015.
- ^ Prelas MA, Popovici G, Bigelow LK (1998). Handbook of industrial diamonds and diamond films. CRC Press. pp. 984–992. ISBN 978-0-8247-9994-6.
- ^ «Gem Cutting». Popular Mechanics. 74 (5): 760–764. 1940. ISSN 0032-4558.
- ^ Rapaport M. «Keep the Diamond Dream Alive». Rapaport Magazine. Diamonds.net. Retrieved September 9, 2012.
- ^ a b JCK Staff (January 26, 2011). «10 Things Rocking the Industry». JCK. Jckonline.com. Archived from the original on January 7, 2013. Retrieved September 9, 2012.
- ^ a b Epstein EJ (1982). «Have You Ever Tried To Sell a Diamond?». The Atlantic. Retrieved May 5, 2009.
- ^ Bates R (January 14, 2011). «Interview with Forevermark CEO». JCK. Jckonline.com. Archived from the original on November 28, 2012. Retrieved September 9, 2012.
- ^ Harlow GE (1998). The nature of diamonds. Cambridge University Press. p. 34. ISBN 978-0-521-62935-5.
- ^ Kogel JE (2006). Industrial minerals & rocks. Society for Mining, Metallurgy, and Exploration (U.S.). p. 416. ISBN 978-0-87335-233-8.
- ^ «The Australian Diamond Industry». Archived from the original on July 16, 2009. Retrieved August 4, 2009.
- ^ Erlich E, Hausel DW (2002). Diamond deposits: origin, exploration, and history of discovery. SME. p. 158. ISBN 978-0-87335-213-0.
- ^ «Diamond: The mineral Diamond information and pictures». minerals.net. Retrieved September 24, 2014.
- ^ a b c «Industrial Diamonds Statistics and Information». United States Geological Survey. Retrieved May 5, 2009.
- ^ a b Spear KE, Dismukes JP (1994). Synthetic Diamond: Emerging CVD Science and Technology. Wiley–IEEE. p. 628. ISBN 978-0-471-53589-8.
- ^ Holtzapffel C (1856). Turning And Mechanical Manipulation. Holtzapffel & Co. pp. 176–178. ISBN 978-1-879335-39-4.
- ^ Coelho RT, Yamada S, Aspinwall DK, Wise ML (1995). «The application of polycrystalline diamond (PCD) tool materials when drilling and reaming aluminum-based alloys including MMC». International Journal of Machine Tools and Manufacture. 35 (5): 761–774. doi:10.1016/0890-6955(95)93044-7.
- ^ Sakamoto M, Endriz JG, Scifres DR (1992). «120 W CW output power from monolithic AlGaAs (800 nm) laser diode array mounted on diamond heatsink». Electronics Letters. 28 (2): 197–199. Bibcode:1992ElL….28..197S. doi:10.1049/el:19920123.
- ^ a b Yarnell A (2004). «The Many Facets of Man-Made Diamonds». Chemical and Engineering News. 82 (5): 26–31. doi:10.1021/cen-v082n005.p026.
- ^ a b «Conflict Diamonds». United Nations. March 21, 2001. Archived from the original on March 9, 2010. Retrieved May 5, 2009.
- ^ Catelle WR (1911). The Diamond. John Lane Co. p. 159.
- ^ a b c Hershey W (1940). The Book of Diamonds. New York: Hearthside Press. pp. 22–28. ISBN 978-1-4179-7715-4.
- ^ Ball V (1881). «1». Diamonds, Gold and Coal of India. London: Trübner & Co. p. 1. Ball was a geologist in British service.
- ^ «Biggest diamond found in Panna». Mail Today. July 1, 2010. Archived from the original on July 7, 2011.
- ^ Shillington K (2005). Encyclopedia of African history. CRC Press. p. 767. ISBN 978-1-57958-453-5.
- ^ a b Janse AJ (2007). «Global Rough Diamond Production Since 1870». Gems & Gemology. 43 (2): 98–119. doi:10.5741/GEMS.43.2.98.
- ^ a b Lorenz V (2007). «Argyle in Western Australia: The world’s richest diamantiferous pipe; its past and future». Gemmologie, Zeitschrift der Deutschen Gemmologischen Gesellschaft. 56 (1–2): 35–40.
- ^ a b Cooke S (October 17, 2004). «Microscopic diamond found in Montana». The Montana Standard. Archived from the original on January 21, 2005. Retrieved May 5, 2009.
- ^ Marshall S, Shore J (2004). «The Diamond Life». Guerrilla News Network. Archived from the original on January 26, 2007. Retrieved March 21, 2007.
- ^ Shigley JE, Chapman J, Ellison RK (2001). «Discovery and Mining of the Argyle Diamond Deposit, Australia» (PDF). Gems & Gemology. 37 (1): 26–41. doi:10.5741/GEMS.37.1.26. Archived from the original (PDF) on September 30, 2009. Retrieved February 20, 2010.
- ^ a b Basedau M, Mehler A (2005). Resource politics in Sub-Saharan Africa. GIGA-Hamburg. pp. 305–313. ISBN 978-3-928049-91-7.
- ^ World Federation of Diamond Bourses (WFDB) and International Diamond Manufacturers Association: Joint Resolution of 19 July 2000. World Diamond Council. July 19, 2000. ISBN 978-90-04-13656-4. Retrieved November 5, 2006.
- ^ «Voluntary Code of Conduct For Authenticating Canadian Diamond Claims» (PDF). Canadian Diamond Code Committee. 2006. Archived from the original (PDF) on February 29, 2012. Retrieved October 30, 2007.
- ^ Kjarsgaard BA, Levinson AA (2002). «Diamonds in Canada». Gems and Gemology. 38 (3): 208–238. doi:10.5741/GEMS.38.3.208.
- ^ A meta-analysis of the environmental impact specific to diamond mining is in Oluleye G. Environmental Impacts of Mined Diamonds (PDF) (Report). Imperial College London Consultants. Archived (PDF) from the original on 2021-12-03. Retrieved 2022-07-01.
- ^ a b c «The Global Diamond Industry: Lifting the Veil of Mystery» (PDF). Bain & Company. Archived (PDF) from the original on 2012-01-31. Retrieved January 14, 2012.
- ^ Shigley JE, Abbaschian R (2002). «Gemesis Laboratory Created Diamonds». Gems & Gemology. 38 (4): 301–309. doi:10.5741/GEMS.38.4.301.
- ^ Shigley JE, Shen AH, Breeding CM, McClure SF, Shigley JE (2004). «Lab Grown Colored Diamonds from Chatham Created Gems». Gems & Gemology. 40 (2): 128–145. doi:10.5741/GEMS.40.2.128.
- ^ Werner M, Locher R (1998). «Growth and application of undoped and doped diamond films». Reports on Progress in Physics. 61 (12): 1665–1710. Bibcode:1998RPPh…61.1665W. doi:10.1088/0034-4885/61/12/002. S2CID 250878100.
- ^ Pisani B (August 27, 2012). «The Business of Diamonds, From Mining to Retail». CNBC.
- ^ Kogel JE (2006). Industrial Minerals & Rocks. SME. pp. 426–430. ISBN 978-0-87335-233-8.
- ^ O’Donoghue M, Joyner L (2003). Identification of gemstones. Great Britain: Butterworth-Heinemann. pp. 12–19. ISBN 978-0-7506-5512-5.
- ^ Barnard AS (2000). The diamond formula. Butterworth-Heinemann. p. 115. ISBN 978-0-7506-4244-6.
- ^ Shigley JE (2007). «Observations on new coated gemstones». Gemmologie: Zeitschrift der Deutschen Gemmologischen Gesellschaft. 56 (1–2): 53–56.
- ^ US 4488821, Wenckus JF, «Method and means of rapidly distinguishing a simulated diamond from natural diamond», published December 18, 1984, assigned to Ceres Electronics Corporation; U.S. Patent 4,488,821
- ^ a b Edwards HG, Chalmers GM (2005). Raman spectroscopy in archaeology and art history. Royal Society of Chemistry. pp. 387–394. ISBN 978-0-85404-522-8.
- ^ a b Welbourn C (2006). «Identification of Synthetic Diamonds: Present Status and Future Developments, Proceedings of the 4th International Gemological Symposium». Gems and Gemology. 42 (3): 34–35.
- ^ Donahue PJ (April 19, 2004). «DTC Appoints GIA Distributor of DiamondSure and DiamondView». Professional Jeweler Magazine. Retrieved March 2, 2009.
- ^ «SSEF diamond spotter and SSEF illuminator». SSEF Swiss Gemmological Institute. Archived from the original on June 27, 2009. Retrieved May 5, 2009.
- ^ Liddell HG, Scott R. «Adamas». A Greek-English Lexicon. Perseus Project.
- ^ Pliny the Elder (2004). Natural History: A Selection. Penguin Books. p. 371. ISBN 978-0-14-044413-1.
- ^ «Chinese made first use of diamond». BBC News. May 17, 2005. Retrieved March 21, 2007.
- ^ See:
- Lavoisier A (2007-10-15) [1772 (part 2)], «Premier mémoire sur la destruction du diamant par le feu» [First memoir on the destruction of diamond by fire], Histoire de l’Académie royale des sciences, avec les Mémoires de Mathématique & de Physique, tirés des registres de cette Académie [History of the Royal Academy of Sciences, with the Memoirs of Mathematics & Physics, drawn from the records of this academy]] (in French), Gallica: Académie des sciences, pp. 564–591, ISSN 1967-4783, ark:/12148/bpt6k35711, retrieved 2022-07-01
- Lavoisier A (2007-10-15) [1772 (part 2)], «Second mémoire sur la destruction du diamant par le feu» [Second memoir on the destruction of diamond by fire], Histoire de l’Académie royale des sciences, avec les Mémoires de Mathématique & de Physique, tirés des registres de cette Académie (in French), Gallica: Académie des sciences, pp. 591–616, ISSN 1967-4783, ark:/12148/bpt6k35711, retrieved 2022-07-01
- ^ Smithson T (1797) [December 15, 1797]. «On the nature of the diamond». Philosophical Transactions of the Royal Society of London: Giving Some Account of the Present Undertakings, Studies, and Labours of the Ingenious, in Many Considerable Parts of the World. 87: 123–127. doi:10.1098/rstl.1797.0005. S2CID 186213726. Retrieved 2022-07-01.
General and cited references
- Even-Zohar C (2007). From Mine to Mistress: Corporate Strategies and Government Policies in the International Diamond Industry (2nd ed.). Mining Journal Press.
- Davies G (1994). Properties and growth of diamond. INSPEC. ISBN 978-0-85296-875-8.
- O’Donoghue M (2006). Gems. Elsevier. ISBN 978-0-7506-5856-0.
- O’Donoghue M, Joyner L (2003). Identification of gemstones. Great Britain: Butterworth-Heinemann. ISBN 978-0-7506-5512-5.
- Feldman A, Robins LH (1991). Applications of Diamond Films and Related Materials. Elsevier. ISBN 978-1-48329124-6.
- Field JE (1979). The Properties of Diamond. London: Academic Press. ISBN 978-0-12-255350-9.
- Field JE (1992). The Properties of Natural and Synthetic Diamond. London: Academic Press. ISBN 978-0-12-255352-3.
- Hershey W (1940). The Book of Diamonds. Hearthside Press New York. ISBN 978-1-4179-7715-4.
- Koizumi S, Nebel CE, Nesladek M (2008). Physics and Applications of CVD Diamond. Wiley VCH. ISBN 978-3-527-40801-6.
- Pan LS, Kani DR (1995). Diamond: Electronic Properties and Applications. Kluwer Academic Publishers. ISBN 978-0-7923-9524-9.
- Pagel-Theisen V (2001). Diamond Grading ABC: the Manual. Antwerp: Rubin & Son. ISBN 978-3-9800434-6-5.
- Radovic RL, Walker RM, Thrower PA (1965). Chemistry and physics of carbon: a series of advances. New York: Marcel Dekker. ISBN 978-0-8247-0987-7.
- Tolkowsky M (1919). Diamond Design: A Study of the Reflection and Refraction of Light in a Diamond. London: E. & F.N. Spon.
- Wise RW (2016). Secrets of the Gem Trade: The Connoisseur’s Guide to Precious Gemstones (Second ed.). Brunswick House Press. ISBN 978-0-9728223-2-9.
- Zaitsev AM (2001). Optical Properties of Diamond: A Data Handbook. Springer. ISBN 978-3-540-66582-3.
Further reading
- Epstein EJ (February 1982). «Have You Ever Tried to Sell a Diamond?». The Atlantic Monthly. Archived from the original on 15 March 2006. Retrieved 2 January 2023.
- Tyson P (November 2000). «Diamonds in the Sky». «The Diamond Deception». Nova. PBS. Retrieved 2 January 2023.
External links
- Properties of diamond: Ioffe database
- «A Contribution to the Understanding of Blue Fluorescence on the Appearance of Diamonds» at the Wayback Machine (archived 2017-09-08) (2007) Gemological Institute of America (GIA)
Углерод | |
---|---|
Атомный номер | 6 |
Внешний вид простого вещества | ![]() матово-черный (графит), либо прозрачный (алмаз) |
Свойства атома | |
Атомная масса (молярная масса) | 12,011 (природная смесь изотопов) а.е.м. (г/моль) |
Радиус атома | 91 пм |
Энергия ионизации (первый электрон) | 1085,7 (11,25) кДж/моль (эВ) |
Электронная конфигурация | [He] 2s2 2p2 |
Химические свойства | |
Ковалентный радиус | 77 пм |
Радиус иона | 16 (+4e) 260 (-4e) пм |
Электроотрицательность (по Полингу) | 2,55 |
Электродный потенциал | — |
Степени окисления | -4, -2, 2, 4 |
Термодинамические свойства простого вещества | |
Плотность | 2,25 (графит) г/см³ |
Молярная теплоёмкость | 8,54 (графит) Дж/(K·моль) |
Теплопроводность | 1,59 Вт/(м·K) |
Температура плавления | 3 820 K |
Теплота плавления | n/a кДж/моль |
Температура кипения | 5 100 K |
Теплота испарения | n/a кДж/моль |
Молярный объём | 5,3 см³/моль |
Кристаллическая решётка простого вещества | |
Структура решётки | гексагональная (графит), кубическая (алмаз) |
Параметры решётки | a=2,46; c=6,71 (графит); а=3,567 (алмаз) Å |
Отношение c/a | 2,73 (графит) |
Температура Дебая | 1860 (алмаз) K |
C | 6 |
12,0107 | |
[He]2s22p2 | |
Углерод |
Углерод (химический символ C) химический элемент 4-ой группы главной подгруппы 2-го периода периодической системы Менделеева, порядковый номер 6, атомная масса природной смеси изотопов 12,0107 г/моль.
История
Схема атома углерода
Углерод в виде древесного угля применялся в глубокой древности для выплавки металлов. Издавна известны аллотропные модификации углерода— алмаз и графит. Элементарная природа углерода установлена А. Лавуазье в конце 1780-х годов.
Происхождение названия
Международное название: carbō — уголь.
Физические свойства
Углерод существует во множестве аллотропных модификаций с очень разнообразными физическими свойствами. Разнообразие модификаций обусловлено способностью углерода образовывать химические связи разного типа.
Изотопы углерода
Природный углерод состоит из двух стабильных изотопов— 12С (98,892%) и 13С (1,108%) и одного радиоактивного изотопа 14С (β-излучатель, Т½= 5730 лет), сосредоточенного в атмосфере и верхней части земной коры. Он постоянно образуется в нижних слоях стратосферы в результате воздействия нейтронов космического излучения на ядра азота по реакции: 14N (n, p) 14C, а также, с середины 1950-х годов, как техногенный продукт работы АЭС и в результате испытания водородных бомб.
На образовании и распаде 14С основан метод радиоуглеродного датирования, широко применяющийся в четвертичной геологии и археологии.
Аллотропные модификации углерода
Упрощенная фазовая диаграмма углерода, заштрихованы области где аллотропные модификации могут быть метастабильны. (diamond— алмаз, graphite— графит, liquid— жидкость, vapor— газ)
Схемы строения различных модификаций углерода
a: алмаз, b: графит, c: лонсдейлит
d: фуллерен— букибол C60, e: фуллерен C540, f: фуллерен C70
g: аморфный углерод, h: углеродная нанотрубка
- Аллотропия углерода
графит
алмаз
карбин
лонсдейлит
фуллерены
углеродные нанотрубки
графен
аморфный углерод
- уголь
- техуглерод
- сажа
Электронные орбитали атома углерода могут иметь различную геометрию, в зависимости от степени гибридизации его электронных орбиталей. Существует три основных геометрии атома углерода.
Тетраэдрическая — образуется при смешении одного s- и трех p-электронов (sp3-гибридизация). Атом углерода находится в центре тетраэдра, связан четырьмя эквивалентными σ-связями с атомами углерода или иными в вершинах тетраэдра. Такой геометрии атома углерода соответствуют аллотропные модификации углерода алмаз и лонсдейлит. Такой гибридизацией обладает углерод, например, в метане и других углеводородах.
Тригональная — образуется при смешении одной s- и двух p-электронных орбиталей (sp²-гибридизация). Атом углерода имеет три равноценные σ-связи, расположенные в одной плоскости под углом 120° друг к другу. Не участвующая в гибридизации p-орбиталь, расположенная перпендикулярно плоскости σ-связей, используется для образования π-связи с другими атомами. Такая геометрия углерода характерна для графита, фенола и др.
Дигональная — образуется при смешении одного s- и одного p-электронов (sp-гибридизация). При этом два электронных облака вытянуты вдоль одного направления и имеют вид несимметричных гантелей. Два других р-электрона дают π-связи. Углерод с такой геометрией атома образует особую аллотропную модификацию — карбин.
Графит и алмаз
Основные и хорошо изученные кристаллические модификации углерода— алмаз и графит. При нормальных условиях термодинамически устойчив только графит, а алмаз и другие формы метастабильны. При атмосферном давлении и температуре выше 1200 Kалмаз начинает переходить в графит, выше 2100 Kпревращение совершается за секунды. ΔН0 перехода— 1,898 кДж/моль. При нормальном давлении углерод сублимируется при 3780 K. Жидкий углерод существует только при определенном внешнем давлении. Тройные точки: графит-жидкость-пар Т =4130 K, р =10,7 МПа. Прямой переход графита в алмаз происходит при 3000 Kи давлении 11—12 ГПа.
При давлении свыше 60 ГПа предполагают образование весьма плотной модификации С III (плотность на 15—20% выше плотности алмаза), имеющей металлическую проводимость. При высоких давлениях и относительно низких температурах (ок. 1200 K) из высокоориентированного графита образуется гексагональная модификация углерода с кристаллической решеткой типа вюрцита— лонсдейлит (а =0,252 нм, с =0,412 нм, пространственная группа Р63/ттс), плотность 3,51 г/см³, то есть такая же, как у алмаза. Лонсдейлит найден также в метеоритах.
Ультрадисперсные алмазы (наноалмазы)
В 1980-е гг. в СССР было обнаружено, что в условиях динамического нагружения углеродсодержащих материалов могут образовываться алмазоподобные структуры, получившие название ультрадисперсных алмазов (УДА). В настоящее время всё чаще применяется термин «наноалмазы». Размер частиц в таких матералах составляет единицы нанометров. Условия образования УДА могут быть реализованы при детонации взрывчатых веществ с значительным отрицательным кислородным балансом, например смесей тротила с гексогеном. Такие условия могут быть реализованы также при ударах небесных тел о поверхность Земли в присутствии углеродсодержащих материалов (органика, торф, уголь и пр.). Так, в зоне падения Тунгусского метеорита в лесной подстилке были обнаружены УДА.
Карбин
Кристаллическая модификация углерода гексагональной сингонии с цепочечным строением молекул называется карбин. Цепи имеют либо полиеновое строение (—C≡C—), либо поликумуленовое (=C=C=). Известно несколько форм карбина, отличающихся числом атомов в элементарной ячейке, размерами ячеек и плотностью (2,68—3,30 г/см³). Карбин встречается в природе в виде минерала чаоита (белые прожилки и вкрапления в графите) и получен искусственно— окислительной дегидрополиконденсацией ацетилена, действием лазерного излучения на графит, из углеводородов или CCl4 в низкотемпературной плазме.
Карбин представляет собой мелкокристаллический порошок чёрного цвета (плотность 1,9-2 г/см³), обладает полупроводниковыми свойствами. Получен в искусственных условиях из длинных цепочек атомов углерода, уложенных параллельно друг другу.
Карбин— линейный полимер углерода. В молекуле карбина атомы углерода соединены в цепочки поочередно либо тройными и одинарными связями (полиеновое строение), либо постоянно двойными связями (поликумуленовое строение). Это вещество впервые получено советскими химиками В.В.Коршаком, А.М.Сладковым, В.И.Касаточкиным и Ю.П.Кудрявцевым в начале 60-х гг. в Институте элементоорганических соединений Академии наук СССР .Карбин обладает полупроводниковыми свойствами, причём под воздействием света его проводимость сильно увеличивается. На этом свойстве основано первое практическое применение— в фотоэлементах.
Фуллерены и углеродные нанотрубки
Углерод известен также в виде кластерных частиц С60, С70, C80, C90, C100 и подобных (фуллерены), а также графенов и нанотрубок.
Аморфный углерод
В основе строения аморфного углерода лежит разупорядоченная структура монокристаллического (всегда содержит примеси) графита. Это кокс, бурые и каменные угли, техуглерод, сажа, активный уголь.
Нахождение в природе
Содержание углерода в земной коре 0,1% по массе. Свободный углерод находится в природе в виде алмаза и графита. Основная масса углерода в виде природных карбонатов (известняки и доломиты), горючих ископаемых— антрацит (94—97% С), бурые угли (64—80% С), каменные угли (76—95% С), горючие сланцы (56—78% С), нефть (82—87% С), горючих природных газов (до 99% метана), торф (53—56% С), а также битумы и др. В атмосфере и гидросфере находится в виде диоксида углерода СО2, в воздухе 0,046% СО2 по массе, в водах рек, морей и океанов в ~60 раз больше. Углерод входит в состав растений и животных (~18%).
В организм человека углерод поступает с пищей (в норме около 300 г в сутки). Общее содержание углерода в организме человека достигает около 21% (15кг на 70кг массы тела). Углерод составляет 2/3 массы мышц и 1/3 массы костной ткани. Выводится из организма преимущественно с выдыхаемым воздухом (углекислый газ) и мочой (мочевина)
Кругооборот углерода в природе включает биологический цикл, выделение СО2 в атмосферу при сгорании ископаемого топлива, из вулканических газов, горячих минеральных источников, из поверхностных слоев океанических вод и др. Биологический цикл состоит в том, что углерод в виде СО2 поглощается из тропосферы растениями. Затем из биосферы вновь возвращается в геосферу: с растениями углерод попадает в организм животных и человека, а затем при гниении животных и растительных материалов— в почву и в виде СО2— в атмосферу.В парообразном состоянии и в виде соединений с азотом и водородом углерод обнаружен в атмосфере Солнца, планет, он найден в каменных и железных метеоритах.
Большинство соединений углерода, и прежде всего углеводороды, обладают ярко выраженным характером ковалентных соединений. Прочность простых, двойных и тройных связей атомов С между собой, способность образовывать устойчивые цепи и циклы из атомов С обусловливают существования огромного числа углеродсодержащих соединений, изучаемых органической химией.
Химические свойства
При обычных температурах углерод химически инертен, при достаточно высоких соединяется со многими элементами, проявляет сильные восстановительные свойства. Химическая активность разных форм углерода убывает в ряду: аморфный углерод, графит, алмаз, на воздухе они воспламеняются при температурах соответственно выше 300—500 °C, 600—700 °C и 850—1000 °C.
Степени окисления +4, −4, редко +2 (СО, карбиды металлов), +3 (C2N2, галогенцианы); сродство к электрону 1,27 эВ; энергия ионизации при последовательном переходе от С0 к С4+ соответственно 11,2604, 24,383, 47,871 и 64,19 эВ.
Неорганические соединения
Углерод реагирует со многими элементами с образованием карбидов.
Продукты горения— оксид углерода CO и диоксид углерода СО2. Известен также неустойчивый оксид С3О2 (температура плавления −111°C, температура кипения 7°C) и некоторые другие оксиды. Графит и аморфный углерод начинают реагировать с Н2 при 1200°C, с F2— соответственно 900°C.
CO2 с водой образует слабую угольную кислоту— H2CO3, которая образует соли— Карбонаты. На Земле наиболее широко распространены карбонаты кальция (мел, мрамор, кальцит, известняк и др. минералы) и магния (доломит).
Графит с галогенами, щелочными металлами и др. веществами образует соединения включения. При пропускании электрического разряда между угольными электродами в среде N2 образуется циан, при высоких температурах взаимодействием углерода со смесью Н2 и N2 получают синильную кислоту. С серой углерод дает сероуглерод CS2, известны также CS и C3S2. С большинством металлов, бором и кремнием углерод образует карбиды. Важна в промышленности реакция углерода с водяным паром: С +Н2О =СО +Н2 (Газификация твердых топлив). При нагревании углерод восстанавливает оксиды металлов до металлов, что широко используется в металлургии.
Органические соединения
Благодаря способности углерода образовывать полимерные цепочки, существует огромный класс соединений на основе углерода, которых значительно больше, чем неорганических, и изучением которых занимается органическая химия. Среди них наиболее обширные группы: углеводороды, белки, жиры и др.
Соединения углерода составляют основу земной жизни, а их свойства во многом определяют спектр условий, в которых подобные формы жизни могут существовать. По числу атомов в живых клетках доля углерода около 25%, по массовой доле— около 18%.
Применение
Графит используется в карандашной промышленности. Также его используют в качестве смазки при особо высоких или низких температурах.
Алмаз, благодаря исключительной твердости, незаменимый абразивный материал. Алмазным напылением обладают шлифовальные насадки бормашин. Кроме этого, ограненные алмазы — бриллианты используются в качестве драгоценных камней в ювелирных украшениях. Благодаря редкости, высоким декоративным качествам и стечению исторических обстоятельств, бриллиант неизменно является самым дорогим драгоценным камнем. Исключительно высокая теплопроводность алмаза (до 2000 Вт/м•К) делает его перспективным материалом для полупроводниковой техники в качестве подложек для процессоров. Но относительно высокая цена (около 50 долларов/грамм) и сложность обработки алмаза ограничивают его применение в этой области.
В фармакологии и медицине широко используются различные соединения углерода— производные угольной кислоты и карбоновых кислот, различные гетероциклы, полимеры и другие соединения. Так, карболен (активированный уголь), применяется для абсорбции и выведения из организма различных токсинов; графит (в виде мазей)— для лечения кожных заболеваний; радиоактивные изотопы углерода— для научных исследований (радиоуглеродный анализ).Углерод играет огромную роль в жизни человека. Его применения столь же разнообразны, как сам этот многоликий элемент.
Углерод является основой всех органических веществ. Любой живой организм состоит в значительной степени из углерода. Углерод— основа жизни. Источником углерода для живых организмов обычно является СО2 из атмосферы или воды. В результате фотосинтеза он попадает в биологические пищевые цепи, в которых живые существа пожирают друг друга или останки друг друга и тем самым добывают углерод для строительства собственного тела. Биологический цикл углерода заканчивается либо окислением и возврашением в атмосферу, либо захоронением в виде угля или нефти.
Углерод в виде ископаемого топлива: угля и углеводородов (нефть, природный газ)— один из важнейших источников энергии для человечества.
Токсическое действие
Углерод входит в состав атмосферных аэрозолей, в результате чего может изменяться региональный климат, уменьшаться количество солнечных дней. Углерод поступает в окружающую среду в виде сажи в составе выхлопных газов автотранспорта, при сжигании угля на ТЭС, при открытых разработках угля, подземной его газификации, получении угольных концентратов и др. Концентрация углерода над источниками горения 100—400 мкг/м³, крупными городами 2,4—15,9 мкг/м³, сельскими районами 0,5— 0,8 мкг/м³. С газоаэрозольными выбросами АЭС в атмосферу поступает (6—15)•109 Бк/сут 14СО2.
Высокое содержание углерода в атмосферных аэрозолях ведет к повышению заболеваемости населения, особенно верхних дыхательных путей и легких. Профессиональные заболевания— в основном антракоз и пылевой бронхит. В воздухе рабочей зоны ПДК, мг/м³: алмаз 8,0, антрацит и кокс 6,0, каменный уголь 10,0, технический углерод и углеродная пыль 4,0; в атмосферном воздухе максимальная разовая 0,15, среднесуточная 0,05 мг/м³.
Токсическое действие 14С, вошедшего в состав молекул белков (особенно в ДНК и РНК), определяется радиационным воздействием бета частиц и ядер отдачи азота (14С (β) → 14N) и трансмутационным эффектом— изменением химического состава молекулы в результате превращения атома С в атом N. Допустимая концентрация 14С в воздухе рабочей зоны ДКА 1,3 Бк/л, в атмосферном воздухе ДКБ 4,4 Бк/л, в воде 3,0•104 Бк/л, предельно допустимое поступление через органы дыхания 3,2•108 Бк/год.
Дополнительная информация
— Соединения углерода
— Радиоуглеродный анализ
— Ортокарбоновая кислота
Аллотропные формы углерода:
Алмаз
Графен
Графит
Карбин
Лонсдейлит
Углеродные нанотрубки
Фуллерены
Аморфные формы:
Сажа
Технический углерод
Уголь
Изотопы углерода:
Нестабильные (менее суток): 8C: Углерод-8, 9C: Углерод-9, 10C: Углерод-10, 11C: Углерод-11
Стабильные: 12C: Углерод-12, 13C: Углерод-13
10—10 000 лет: 14C: Углерод-14
Нестабильные (менее суток): 15C: Углерод-15, 16C: Углерод-16, 17C: Углерод-17, 18C: Углерод-18, 19C: Углерод-19, 20C: Углерод-20, 21C: Углерод-21, 22C: Углерод-22
Таблица нуклидов
Углерод, Carboneum, С (6)
Углерод (англ. Carbon, франц. Carbone, нем. Kohlenstoff) в виде угля, копоти и сажи известен человечеству с незапамятных времен; около 100 тыс. лет назад, когда наши предки овладели огнем, они каждодневно имели дело с углем и сажей. Вероятно, очень рано люди познакомились и с аллотропическими видоизменениями углерода — алмазом и графитом, а также с ископаемым каменным углем. Не удивительно, что горение углеродсодержащих веществ было одним из первых химических процессов, заинтересовавших человека. Так как горящее вещество исчезало, пожираемое огнем, горение рассматривали как процесс разложения вещества, и поэтому уголь (или углерод) не считали элементом. Элементом был огонь — явление, сопровождающее горение; в учениях об элементах древности огонь обычно фигурирует в качестве одного из элементов. На рубеже XVII — XVIII вв. возникла теория флогистона, выдвинутая Бехером и Шталем. Эта теория признавала наличие в каждом горючем теле особого элементарного вещества — невесомого флюида — флогистона, улетучивающегося в процессе горения.
При сгорании большого количества угля остается лишь немного золы, флогистики полагали, что уголь — это почти чистый флогистон. Именно этим объясняли, в частности, «флогистирующее» действие угля, — его способность восстанавливать металлы из «известей» и руд. Позднейшие флогистики, Реомюр, Бергман и др., уже начали понимать, что уголь представляет собой элементарное вещество. Однако впервые таковым «чистый уголь» был признан Лавуазье, исследовавшим процесс сжигания в воздухе и кислороде угля и других веществ. В книге Гитона де Морво, Лавуазье, Бертолле и Фуркруа «Метод химической номенклатуры» (1787) появилось название «углерода» (carbone) вместо французского «чистый уголь» (charbone pur). Под этим же названием углерод фигурирует в «Таблице простых тел» в «Элементарном учебнике химии» Лавуазье. В 1791 г. английский химик Теннант первым получил свободный углерод; он пропускал пары фосфора над прокаленным мелом, в результате чего образовывался фосфат кальция и углерод. То, что алмаз при сильном нагревании сгорает без остатка, было известно давно. Еще в 1751 г. французский король Франц I согласился дать алмаз и рубин для опытов по сжиганию, после чего эти опыты даже вошли в моду. Оказалось, что сгорает лишь алмаз, а рубин (окись алюминия с примесью хрома) выдерживает без повреждения длительное нагревание в фокусе зажигательной линзы. Лавуазье поставил новый опыт по сжиганию алмаза с помощью большой зажигательной машины, пришел к выводу, что алмаз представляет собой кристаллический углерод. Второй аллотроп углерода — графит в алхимическом периоде считался видоизмененным свинцовым блеском и назывался plumbago; только в 1740 г. Потт обнаружил отсутствие в графите какой-либо примеси свинца. Шееле исследовал графит (1779) и будучи флогистиком счел его сернистым телом особого рода, особым минеральным углем, содержащим связанную «воздушную кислоту» (СО2,) и большое количество флогистона.
Двадцать лет спустя Гитон де Морво путем осторожного нагревания превратил алмаз в графит, а затем в угольную кислоту.
Международное название Carboneum происходит от лат. carbo (уголь). Слово это очень древнего происхождения. Его сопоставляют с cremare — гореть; корень саг, cal, русское гар, гал, гол, санскритское ста означает кипятить, варить. Со словом «carbo» связаны названия углерода и на других европейских языках (carbon, charbone и др.). Немецкое Kohlenstoff происходит от Kohle — уголь (старогерманское kolo, шведское kylla — нагревать). Древнерусское угорати, или угарати (обжигать, опалять) имеет корень гар, или гор, с возможным переходом в гол; уголь по-древнерусски югъль, или угъль, того же происхождения. Слово алмаз (Diamante) происходит от древнегреческого — несокрушимый, непреклонный, твердый, а графит от греческого — пишу.
В начале XIX в. старое слово уголь в русской химической литературе иногда заменялось словом «углетвор» (Шерер, 1807; Севергин, 1815); с 1824 г. Соловьев ввел название углерод.
На чтение 5 мин Просмотров 6.3к.
Содержание
- Состав, формула и свойства алмазов
- Формула вещества
- Формула алмаза
- Химические свойства алмаза
Алмаз — это один из самых прекрасных и дорогих минералов на планете, завоевавший множество поклонников. Приоритетным свойством камня выступает его твердость, которая является самым высоким показателем среди всех минералов.
Состав, формула и свойства алмазов
Поэтому многим интересно, как образуется камень, какая формула алмаза и можно ли его вырастить в лаборатории. Ученые по-прежнему после столетия опытов не могут ответить на все вопросы с точностью, поскольку в некоторых ситуациях камень ведет себя аномально.
Формула вещества
Состоит алмаз полностью из углерода. Этого элемента содержится около 0,15% в Земной коре. Атомный номер вещества — 6, что говорит о количестве протонов в ядре. Соответственно, у алмазов, которые полностью состоят из углерода, то есть являются аллотропной формой этого вещества, атомный номер такой же.
Такое понятие, как форма аллотропной модификации, означает, что из простого вещества, например, углерода, могут формироваться другие простые вещества, которые будут отличаться между собой свойствами и строением атомов.
То есть содержание одно, а форма и вид — абсолютно разные, взять хотя бы противоположности: графит и алмаз. При этом углерод — один из немногих веществ, которое имеет несколько форм модификаций.
Выделяют такие вещества, которые состоят только из углерода:
- алмаз;
- графит;
- карбин;
- лонсдейлит;
- фуллерены;
- углеродные нанотрубки для изготовления микроволокон;
- графен;
- уголь, сажа.
Интересным вопросом для ученых является вопрос, можно ли превратить одну аллотропную модификацию в другую. Как раз этим они и занимаются по отношению к графиту и другим веществам из этой группы.
Поскольку стоимость алмаза — самая высокая, а цена других модификаций ниже. Пока процесс возможен только лишь в обратную сторону: если алмаз нагреть без воздуха до температуры свыше 500 градусов по Цельсию, то камень взорвется и превратится в графит.
При этом дальнейшее плавление камня показывает аномальные результаты, отличные от других веществ. А вот в обратном направлении реакция не происходит.
Разница между модификациями объясняется строением кристаллической решетки вещества. Химическая формула тут не играет никакой роли. Вся суть заключается в пространственной конфигурации атомов углерода и связи между ними. Так, в строении алмаза решетка имеет кубическое строение.
Связь между атомами — самая прочная, с точки зрения химии, ковалентная. При этом кубическая система использует всего 18 атомов и считается самой плотной формой упаковки этих частиц. Поэтому алмаз и является самым твердым веществом планеты.
В центрах граней тетраэдра также располагаются атомы, связанные между собой ковалентно. А вот если рассматривать тот же графит, то в его кристаллической решетке часть связей ковалентная, а часть дисульфидная, которая имеет свойство разрываться. Вследствие этого электроны могут мигрировать, и вещество получает металлические свойства.
Но это разбор атомных форм углерода, поскольку именно атомы и формируют кристаллическую решетку. А вот недавно ученые обнаружили и молекулярные формы вещества в составе фуллеренов, многогранников из углерода. Сейчас обнаружили новые молекулярные соединения с содержанием углерода — от С60 до С540, над которыми проводятся исследования.
На основе формулы, а также конфигурации атомов, ученые пытаются воссоздать картину в лабораторных условиях. В природе алмазы находят в кимберлитовых и лампроитовых трубках, а также на россыпях. Камни формируются миллионы лет в определенных условиях с участием магматических пород, сейсмоактивности, а также под действием высоких температур.
Существует и версия о занесении алмаза вместе с метеоритами, поскольку углерода достаточно много в космическом пространстве. А также одну из его модификаций — лосдейлит — ученые обнаружили в составе метеоритов.
На сегодняшний день алмазы изготавливают такими способами:
- Под давлением и высокой температурой в специальных машинах. Ученые добиваются от графита образования новых ковалентных связей. Такой тип камней называют HPHT.
- Пленочный способ происходит также с участием графита, осаждение которого происходит под действием паров метана.
- Производство камней вследствие взрывного синтеза.
Даже несмотря на то, что все знают формулу бриллианта, ну или его не ограненного вещества (алмаза), никто из ученых не смог с точностью воспроизвести камень с таким же строением кристаллической решетки, как это сделала природа. Поэтому стоимость камня держится на высоком уровне, а добыча из недр Земли не прекращается.
Формула алмаза
Физические свойства – это одно из самых твёрдых веществ с плотностью 3,47—3,55 г/см, обычно бесцветный, но может иметь различные цвета, прозрачный, хрупкий, блестящий.
Плохо проводит электрический ток. Не плавится, сублимируется при 3506,85°С, устойчив при нагревании в отсутствие кислорода.
Кристаллическая решетка алмаза гранецентрированная кубическая (а = 0,357 нм, z = 4). Атомы углерода в алмазе имеют
-гибридизацию. Каждый атом С в структуре алмаза находится в центре тетраэдра, вершинами которого служат четыре соседних атома.
На рисунке ниже показана элементарная ячейка алмаза:
Химические свойства алмаза
Так как алмаз очень твёрдое вещество, то он является достаточно инертным, поэтому его основная реакция – это горение в кислороде при высокой температуре:
Источники:
https://okaratah.com/dragocennye/almaz/formula-almaza.html
http://ru.solverbook.com/spravochnik/formuly-po-ximii/formula-almaza/